Top predator status and trends: ecological implications, monitoring and mitigation strategies to promote ecosystem-based management
- 1Marine Biodiversity Protection Unit, Environment Monitoring and Protection and Biodiversity Conservation Department, Italian Institute for Environmental Protection and Research, Rome, Italy
- 2Conservation, Management and Sustainable Use of National Marine Resources Unit, Italian Institute for Environmental Protection and Research, Ozzano dell’Emilia, Italy
- 3Centro de Investigación Mariña, Universidade de Vigo, Future Oceans Lab, Vigo, Spain
- 4Department of Renewable Marine Resources, Institut de Ciències del Mar (ICM-CSIC), Barcelona, Spain
- 5International Estuarine & Coastal Specialists (IECS) Ltd., Leven, United Kingdom
- 6Spanish Institute of Oceanography (IEO-CSIC), Centro Oceanográfico de Málaga, Fuengirola, Spain
- 7Department of Marine Sciences, University of the Aegean, Mytilene, Greece
- 8Stichting Nederlandse Wetenschappelijk Onderzoek Instituten (NIOZ) Royal Netherlands Institute for Sea Research, Department of Coastal Systems, Den Burg, Texel, Netherlands
- 9AZTI, Marine Research, Basque Research and Technology Alliance (BRTA), Sukarrieta, Spain
- 10Wageningen Marine Research, Den Helder, Netherlands
- 11Department of Biology, University of Naples Federico II, Naples, Italy
- 12Department of Evolutionary Biology, Ecology and Environmental Sciences, and Biodiversity Research Institute (IRBio), Faculty of Biology, Universitat de Barcelona, Barcelona, Spain
- 13Conservation, Management and Sustainable Use of National Marine Resources Unit, Italian Institute for Environmental Protection and Research, Chioggia, Italy
- 14Sea Mammal Research Unit, Scottish Oceans Institute, University of St Andrews, St Andrews, United Kingdom
- 15Institute for Marine Biological Resources and Biotechnologies (IRBIM), National Research Council, Mazara del Vallo, Italy
The conservation and management of marine ecosystems hinge on a comprehensive understanding of the status and trends of top predators. This review delves into the ecological significance of marine top predators, examining their roles in maintaining ecosystem stability and functioning through an integrated analysis of current scientific literature. We first assess the efficacy of various monitoring methods, ranging from traditional field observations to cutting-edge technologies like satellite tracking and environmental DNA (eDNA) analysis and evaluating their strengths and limitations in terms of accuracy, spatial coverage, and cost-effectiveness, providing resource managers with essential insights for informed decision-making. Then, by synthesizing data from diverse marine ecosystems, this study offers a comprehensive overview of the trends affecting top predator populations worldwide. We explore the multifaceted impacts of human activities, climate change, and habitat degradation on the abundance and distribution of these key species. In doing so, we shed light on the broader implications of declining top predator populations, such as trophic cascades and altered community structures. Following a thorough assessment of successful strategies for reversing the decline of top predators, a compilation of recommendations is presented, encompassing effective governance interventions. A crucial aspect of effective ecosystem-based management is the implementation of robust monitoring strategies. Mitigation measures are imperative to reverse the adverse impacts on marine top predators. We present a comprehensive array of mitigation options based on successful case studies. These include the establishment of marine protected areas, the enforcement of fisheries regulations, and the promotion of sustainable fishing practices. We deepen the synergies between these strategies and their potential to mitigate human-induced stressors on top predator populations to safeguard their pivotal role in maintaining marine ecosystem structure and function. By examining marine top predators’ ecological significance, analyzing population trends, discussing monitoring techniques, and outlining effective mitigation strategies, we provide a comprehensive resource for researchers, policymakers, and stakeholders engaged in fostering ecosystem-based management approaches. We conclude that integrating these insights into current management frameworks will be essential to safeguard both top predators and the broader marine environment for future generations.
1 Introduction
1.1 Marine top predators in a changing environment
In the Anthropocene Era, marine predators occupying high trophic levels - including some marine mammal, elasmobranch, large teleost, and seabird species - have been reported to be rapidly declining worldwide and are generally assessed as threatened or in poor population conservation status (Ferretti et al., 2008; Phillips et al., 2016; Dulvy et al., 2017; Rodríguez et al., 2019; Burgess and Becker, 2022). In addition to the issue of increasingw extinction risk, top predator populations’ fluctuations have been linked to cascading effects in food webs, behavioral modifications in prey communities, and overall losses of ecosystem functions and services (Myers et al., 2007; Heithaus et al., 2008; Baum and Worm, 2009; Estes et al., 2016).
The main drivers of top predators declines include historical hunting, overfishing, fishery-related bycatch, habitat degradation and loss exacerbated by climate change, prey depletion due to overfishing, invasive species, and other interacting local and global stressors (Pauly et al., 1998; Pauly, 2000; Jackson et al., 2001; Myers and Worm, 2003; Lotze et al., 2006; Ryan et al., 2009; Dias et al., 2019; Ripple et al., 2019; Giménez et al., 2022; Juan-Jordá et al., 2022). In recent years, the escalating climate crisis and the depletion of marine food resources have pushed forward Marine Renewable Energy solutions (e.g., offshore wind farms, offshore hydrogen production, technologies exploiting wave and tidal energy, floating solar photovoltaic energy, etc.) (Borthwick, 2016) and seafood production through mariculture (Campbell et al., 2021). These are important Blue Economy sectors and are generally considered as environmentally friendly. However, they introduce novel and poorly understood stressors on marine ecosystems. If not properly managed or regulated, these emergent ‘uses of the sea’ could contribute to cumulative pressures with significant repercussions on top predator populations and their prey (Bailey et al., 2014).
High trophic-level predator declines have alarmed the scientific community because they compromise the sustainability of whole social-ecological systems. Top predators are instrumental in nutrient cycling, carbon sequestration, habitat engineering, and counterbalancing biological invasions. Their value is also linked to socio-economical aspects, e.g., fishery sustainability, tourism, and bioinspiration (Atwood et al., 2015; Doughty et al., 2016; Haas et al., 2017; Hammerschlag et al., 2019). In addition, marine top predators can be used as sentinels of marine ecosystem status (Hazen et al., 2019; Coll et al., 2019b), and changes in their abundance can act as an early warning of decreasing marine health and trigger species and ecosystem conservation interventions. In this context, our ability to track population trends in marine top predators is key for monitoring the Good Environmental Status (GES) under the European Union Marine Strategy Framework Directive (MSFD; Directive 2008/56/EC) and other similar frameworks (e.g., UN Regional Sea Conventions (RSCs; OSPAR for the north-east Atlantic, HELCOM for the Baltic Sea, Barcelona Convention for the Mediterranean Sea, and Bucharest Convention for the Black Sea), and for informing management actions. For example, 23% of the Indicators of the OSPAR 2023 Quality Status Report on the Northeast Atlantic targeted top predators. See sections 5.1 for a detailed example under the MSFD.
Given all this, conventional sectoral management and piecemeal governance, focusing on a single species or economic sector (e.g., fisheries), is generally seen as an ineffective approach to halting biodiversity loss and securing sustainable use of marine resources. Holistic approaches are necessary to understand ecosystem processes (Pikitch et al., 2004; Curtin and Prellezo, 2010; Long et al., 2015) and enable the conservation of top predators by implementing an ecosystem-based approach. Policy and management strategies need to be informed by a fair understanding of: (i) top predators’ role in ecosystem functioning and services; (ii) the socio-ecological implications of changes in their populations, in particular of processes associated with changes in their abundance and distribution, e.g., due to climate change (driver-pressure-state-impacts) to assess plausible socio-economic scenarios; (iii) conflicts caused by ocean human uses; (iv) management options and tradeoffs costs and effectiveness. This translates into an overall assessment of the costs and benefits of conservation efforts.
Notwithstanding the importance of the holistic approach, dedicated management approaches may be still required for marine top-predator populations and species known to be in very ‘unfavorable conservation status’. The full and/or partial recovery of some top predators, such as for example several species of whales subject to commercial whaling, and of swordfish and tunas subject to large pelagic driftnetting (see examples in Box 1 and section 7) in the 1970-80s, were possible thanks to drastic sectorial measures banning those activities and implementing quotas (UNGA, 1990a, b; UNGA 1992; European Council, 1992; European Council, 1997; European Union, 2019).
Box 1. - The recovery of the Atlantic bluefin tuna
A recent example of recovery, following management measures and favorable environmental conditions, is that of the Atlantic bluefin tuna (Thunnus thynnus) eastern population, a species migrating between the Mediterranean and the eastern Atlantic. In 2007, this bluefin tuna population was considered depleted due to a 60% decline in spawning biomass compared to 1970s levels, a population restructuring toward younger individuals, and predictions of stock collapse (Andrews et al., 2022). In the last two decades, the ICCAT has limited catches by imposing strict quotas (ICCAT, 2017), and strong surveillance of the bluefin tuna fishery has been implemented (Bjørndal, 2021). Such management measures, in combination with several years of favorable environmental conditions for spawning, have led to the recovery of the species to 1970s levels (ICCAT, 2020). However, the recovery of this predator may contribute to conflicts with fisheries targeting small pelagic fish (the main prey of bluefin tuna), which are currently overfished and subject to adverse climate conditions (Coll et al., 2019b; Sbragaglia et al., 2021).
Parallel pathways affecting fisheries-induced changes of shoaling behavior (This figure was published in Sbragaglia et al., 2021, Copyright Elsevier 2021) - Key: Factors affecting directly prey and predator shoaling behaviour: (1) ecological and behavioural traits, demographic and evolutionary processes; (2) fisheries targeting larger shoals. Factors indirectly affecting prey and predator shoaling behaviour: (3) fisheries influencing population density and fish group dynamics. Fishing techniques: (a) pelagic trawls; (b) purse seiners; (c) longliners; (d) recreational fishers; (e) traps/pots fishers.
From a public perspective, there is a relatively limited number of flagship marine top predators. Among them, for example, the charismatic polar bear (Ursus maritimus), the feared killer whales (Orcinus orca), and the great white sharks (Carcharodon carcharias). In this review, we consider ‘top predators’ in a broad sense. These are species that predominantly feed at or near the top of the food web in their ecosystem (upper trophic level consumers) and are relatively free from predation once they reach adult size. Hence, in this review, top predators are not completely free of predation risk, and they may not always occupy the top predator position throughout their life history or across all habitats within their spatial distributions (Sergio et al., 2014).
With a focus on the global policy context, this review critically considers: (i) the existing knowledge on the status and trends of top predators; (ii) the best practices to improve their monitoring, including the potential of novel methods (e.g., eDNA, metabarcoding, biologgers, and remote sensing); (iii) data needs and modeling capacity for assessing the status and trends of top predators; (iv) management options to mitigate their decline in line with the marine biodiversity conservation policy framework.
After reviewing best practices in reversing top predator declines, we provide a set of recommendations on possible effective governance interventions, which would help prevent further declines and rebuild top predator populations.
1.2 Marine ecosystem and international policy framework
For top predators characterized by a large home range or performing migrations, international cooperation is fundamental in identifying and disentangling the underlying causes of changes in distribution and abundance and developing management measures to halt population declines (e.g., ACCOBAMS, 2021; Geelhoed et al., 2022). In 1995 the UN Convention on Biological Diversity (CBD) identified the ‘Ecosystem Approach’ as the main framework for biodiversity protection and sustainable use, from which most Ecosystem-based Management (EBM) terminology derives. This policy also relies on legal principles (e.g., articles 61-67) embedded in the UN Convention on the Law of the Sea (O’Hagan, 2020). The general objective of EBM is sustainable resource exploitation for the benefit of present and future generations (Long et al., 2017). The implementation of the CBD Ecosystem Approach was linked to various strategies, including the 12 Malawi Principles ‘to take effective and urgent action to halt the loss of biodiversity’, the Strategic Plan for Biodiversity 2011–2020, the Aichi Biodiversity Targets and the latest post-2020 global biodiversity framework, which as an ultimate goal in 2050 has that ‘biodiversity is valued, conserved, restored and wisely used, maintaining ecosystem services, sustaining a healthy planet and delivering benefits essential for all people’. EBM recognizes the full array of interactions within an ecosystem, incorporating ecological, economic, social, and cultural perspectives and supporting an adaptive approach tailored to the scale of ecosystems (Katsanevakis et al., 2011). Due to dynamic ecosystems and a chronic lack of comprehensive knowledge of their functioning, the EBM needs to be adaptive (O’Hagan, 2020). In line with these global policies and related initiatives, halting the loss of biodiversity has been one of the key missions of several Regional Seas Conventions (e.g., the Barcelona Convention, OSPAR, HELCOM, etc.), regional Agreements under the Bonn Convention (e.g., Wadden Sea seals Agreement, Agreement on the Conservation of Albatrosses and Petrels, Agreement on the Conservation of Cetaceans of the Black Sea, Mediterranean Sea and Contiguous Atlantic Area, etc.) and regional supranational political and economic inter-governmental entities (e.g., the European Union). Regional commitments and policy tools (e.g., EU MSFD, Barcelona Convention EcAp (Barcelona Convention 2008, 2012, 2013, 2016), EU Maritime Spatial Planning Directive (Directive 2014/89/EU), Barcelona Convention Protocol on Integrated Coastal Zone Management in the Mediterranean (2008), EU Common Fisheries Policy, etc.) have, at least on paper, linked to the concept and ultimate goal of EBM, with contrasting results and some serious inconsistencies (e.g., Berg et al., 2015; O’Hagan, 2020). Such inconsistencies arise from the need for regional legislative and policy frameworks to transition from a sectoral management to more integrated approaches (O’Hagan, 2020). This transition requires (a) a shift in perspective, necessitating both the redesign of frameworks to accommodate additional/new expertise and to expand their geographical scope in terms of shared responsibility, as national jurisdictions are insufficient for ensuring success, and (b) a consistent and clear codification of terminology into legal and policy instruments (e.g., to incorporate the notion of ‘healthy ecosystem’, ‘habitats’, ‘conservation status’, ‘pressure’, ‘state’, ‘impact’, etc.) (Berg et al., 2015; O’Hagan, 2020). The latter is still far from being resolved, and the often ambiguous language in policies allows countries to implement them in rather different ways.
Examples of species and population recovery or stable decline in the Atlantic and Mediterranean Sea (Boxes 1, 2) demonstrate that management measures (or the lack of them) clearly affect the chance to deliver on the CBD’s ultimate goal (i.e., ‘living in harmony with nature’; CBD, 2021). However, these frameworks often employ different monitoring and assessment approaches (e.g., due to the issue of scales, both geographic and temporal, to which legal requirements apply; O’Hagan, 2020), thus applying a holistic framework, such as the ‘Ecosystem Approach’, is a daunting task.
Box 2. - The Mediterranean Sea case
The Mediterranean Sea is a hotspot of both biodiversity (Coll et al., 2010) and human uses and pressures (Coll et al., 2013; Micheli et al., 2013). It has suffered from overexploitation (Tsikliras et al., 2015), destructive fishing (Claudet and Fraschetti, 2010), marine pollution (Danovaro, 2003), including emerging pollutants such as marine litter (Anastasopoulou and Fortibuoni, 2019; Angiolillo and Fortibuoni, 2020; Fossi et al., 2020), global change (Chatzimentor et al., 2023), and invasive species (Tsirintanis et al., 2022). Various EU and regional environmental and conservation policies (e.g., MSFD, Habitats and Birds Directives, EU Biodiversity Strategy, Common Fisheries Policy, Barcelona Convention) aimed to safeguard Mediterranean Biodiversity and the sustainability of marine resources, with varying outcomes.
The Mediterranean monk seal
The conservation of the endemic Mediterranean monk seal, Monachus monachus, is an example of successful conservation efforts in the last decades. Although the species was assessed in 2008 as Critically Endangered with decreasing trend (Aguilar and Lowry, 2010), its global status was recently downgraded first to Endangered (2019) then to Vulnerable (2023), due to an increasing trend in abundance (Karamanlidis et al., 2019, 2023).
Monk seals were historically overexploited for subsistence needs and also killed by fishers due to causing damage to fishing gear and because seals were perceived as competitors for fish. habitat deterioration, coastal development, increased touristic activities, and accidental entanglement in fishing gear also contributed to their dramatic decline (Karamanlidis et al., 2015). By the mid-20th century, the species was eradicated from most of its former range. Since then, it has been protected throughout its range, and conservation measures over the past 30 years have led to an increasing trend in all known subpopulations (Karamanlidis et al., 2015). In all countries with significant monk seal populations, action plans for the conservation of the species have been established, including the protection of essential habitats via MPAs, mitigating interactions with fisheries, improved monitoring, education and public awareness, and rescue and rehabilitation of wounded, sick, and orphaned seals (Karamanlidis et al., 2015). The recent use of eDNA and citizen-science initiatives have offered complementary information on species presence and distribution (Valsecchi et al., 2023).
The case of Audouin’s Gull in the Ebro Delta region
The Audouin’s Gull (Ichthyaetus audouinii, formerly Larus audouinii) in the Ebro Delta region (Western Mediterranean) is an example of both successful management and challenges linked to managing predatory species. The breeding colony in the Ebro Delta showed a rapid growth between the early 1980s and 1990s (Oro and Martinez-Villalta, 1992). This growth can be attributed, in part, to the protection of their breeding area. However, the gulls’ ability to exploit highly abundant and predictable food resources associated with human activities, such as fishing discards, also contributed to this trend (Oro et al., 2013). In fact, Audouin’s gulls from the Ebro Delta have completely adapted their behavior to capitalize on these A’nthropogenic food resources’ (Ouled-Cheikh et al., 2020, 2022). More recently, this colony has faced new challenges because of the arrival of foxes, prompting a substantial number of individuals to disperse to smaller and less accessible colonies (Payo-Payo et al., 2018).
Elasmobranchs
The Mediterranean Sea is a hotspot of extinction risk for sharks and rays (Dulvy et al., 2014). No improvement was observed between the regional International Union for the Conservation of Nature (IUCN) Red List assessments of 2006 and 2016 (Cavanagh and Gibson, 2007; Dulvy et al., 2016).
Indeed, compared to the previous assessment, threatened species increased from 42.3% (2006) to 53.4% (2016), probably due to the significant increase of species included in the CR Category. Pelagic sharks are particularly vulnerable to fishing gear, and the abundance of many species has declined by more than 90%, putting some Mediterranean species at high risk of extinction (Ferretti et al., 2008).
Semi-quantitative analyses of data from FAO, ICCAT, and MEDLEM databases - yielding more than 770 records gathered between 1860 and 2016 from different sources - revealed a significant decline in landings (in both tons and numbers) of some pelagic sharks and rays starting in the early 2000s (Moro et al., 2020). This trend mainly concerns basking sharks (Cetorhinus maximus), blue sharks (Prionace glauca), porbeagles (Lamna nasus), shortfin makos (Isurus oxyrinchus), common threshers (Alopias vulpinus), spinetail devil rays (Mobula mobular) and white sharks, whose negative trend began in the 1970s. Depending on the Mediterranean region, there were between 52% and 96% declines in catches and a contraction of distributions (Moro et al., 2020). The decline in reported catches may be due to a severe population decrease from overexploitation or more responsible fishing practices. Indeed, better enforcement of fishing regulations and banning large driftnets (UNGA, 1990a, b; UNGA 1992) in Mediterranean must have positively affected many marine organisms, including elasmobranchs, over the last decade. This may explain, for example, the increased frequency of sightings of spinetail devil rays (Mancusi et al., 2020), suggesting population recovery. For this reason, this species was considered in an IUCN Green Status assessment (Grace et al., 2022).
The critically endangered Balearic shearwater
The Balearic shearwater (Puffinus mauretanicus) is one of the most endangered seabird species in Europe - classified as Critically Endangered in the IUCN Red List (BirdLife International, 2021). It has a small breeding range and a relatively small population. This species is undergoing an extremely rapid decline, largely related to low adult (and immature) survival rates (BirdLife International, 2021), which is unusually low for a Procellariiform (Oro et al., 2004; Genovart et al., 2016). This is a long-lived species, and therefore the main threats to this species identified are those causing adult mortality.
The greatest threat is fishing bycatch, affecting adults and immatures throughout the species’ range. It is the main driver of the species’ decline, with almost 50% of the mortality caused by this factor (Genovart et al., 2016). Population models predict over 90% decline in three generations with an average extinction time of about 60 years (Genovart et al., 2016). The analyses were based on data from an important colony free of predators, meaning that the average survival rate of the whole population could be even lower (BirdLife International, 2021). Therefore, conservation measures related to reducing mortality in fishing gear are essential for the conservation of the species.
1.3 Effects of top predators on the whole marine ecosystem
The decline of marine top predators (e.g., Box 2) can have diverse and far-reaching ecological consequences. The disruption of food webs is the most studied consequence, as top predators play a crucial role in regulating prey populations (e.g., Ferretti et al., 2010). However, field experiments examining the effects of top predator declines on lower trophic levels have produced varying results, depending on the environment and habitat type (e.g., Heithaus et al., 2008). Declines of marine top predators have been associated with overgrazing, causing a cascade of ecological effects resulting in the loss of ecosystem functions and services (Atwood and Hammill, 2018; Bevilacqua et al., 2021). Such effects can drive regime shifts in coastal systems, leading to biodiversity decline (Guidetti, 2006). Fluctuations in marine top predator abundance can also impact the ecosystem structure; for example, predation loss can boost scavenger populations. Besides an ecosystem top-down control (Aarts et al., 2019), marine top predators contribute to various ecosystem services, such as nutrient cycling, nutrient deposition around their terrestrial sites (for pinnipeds and seabirds), soil formation in polar environments (Şekercioğlu et al., 2004), carbon sequestration, and cultural and recreational services (Roman and McCarthy, 2010).
There are several key examples of ecological consequences of marine top predator decline or loss. In a global analysis, Baum and Worm (2009) reported that declines in large predatory fish, such as sharks and tuna, were associated with changes in prey abundance and diversity and shifts in ecosystem structure and function. The decline in shark abundance at coral reefs caused increases in mesopredator densities and changes in their behavior (Sherman et al., 2020). Similarly, Estes et al. (2009) showed that the decline of sea otters in the Aleutian Islands led to altered behavior and increased abundance of sea urchin, resulting in declines in kelp forests and other ecosystem changes. Along the California coast, the decline of sea otters and sea stars in kelp forests led to changes in prey abundance and diversity, including of sea urchins, crabs, and other invertebrates, which consequently affected the entire ecosystem structure and function (Duffy-Anderson et al., 2019).
The complete removal of top predators from an ecosystem can lead to significant changes in the biomass size spectrum, which can have profound implications for ecosystem function and stability. McCauley et al. (2010) and Atwood et al. 2015 demonstrated that the removal of large predatory fishes, such as groupers and snappers and large sharks, from coral reefs caused a shift towards smaller organism sizes in the biomass size spectra, with an increase in the abundance of small fish and invertebrates and a decrease in the abundance of large predatory fish, leading to deterioration of coral health. The impact of top predator removal on the biomass size spectra may vary depending on the type of ecosystem and the specific predators involved.
2 Monitoring approaches to detect trends of marine top predators
Various techniques are used to monitor abundance trends of marine top predators. These can be divided into ‘direct monitoring methods’ deploying visual and remote sensing tools, and ‘indirect monitoring methods’ using biogeochemical markers, eDNA, biologging, and emerging digital tools. The scope of these approaches depends on the ecological features of the investigated top predators.
2.1 Direct sampling methods to assess trends of top predator distribution and abundance
2.1.1 Scientific trawling surveys
Trawling is one of the most common sampling methods applied to monitor fish, including elasmobranchs, both in fishery-dependent and scientific surveys. Various pelagic and bottom trawls are used to assess species’ presence and estimate their relative abundance (catch per unit effort, CPUE) (Franco et al., 2022). Additional biological variables (e.g., body size, age structure, sex and maturity stage, and stomach content) can often be derived from the catch.
Examples of broad-scale and long-term bottom trawl monitoring programs applying random stratified sampling designs are the International Bottom Trawl Surveys (since 1965) coordinated by the International Council for the Exploration of the Seas (ICES, 2017; ICES, 2020) in the Baltic, North Seas, and adjacent North Atlantic waters and the Mediterranean International Trawl Survey (since 1994) (MEDITS: Spedicato et al., 2019). Data from these monitoring programs have been used to estimate demersal predators’ abundance and distribution (e.g., ICES stock assessments) and to identify the environmental drivers of the population dynamics for some fish species (e.g., Follesa et al., 2019).
2.1.2 Fishery-dependent data
Onboard fishery observations are used to monitor commercially valuable top predators or non-target bycaught species, such as seabirds or marine mammals (e.g., Arcos and Oro, 2002; Louzao et al., 2011a; Field et al., 2013; Louzao et al., 2020). Landing data can also provide valuable information - including species, numbers, weight, and size - albeit with certain limitations. Such data offer broad spatial and temporal coverage of the abundance, distribution, and biological characteristics of fish populations, which can be used to develop conservation management strategies (e.g., Walsh et al., 2009). Onboard observers can help address some of the limitations of fishery-dependent surveys, such as biases resulting from management constraints or intentional misreporting of catches. However, logistic limitations (e.g., non-random sampling) are linked to the intrinsic fisheries nature. At present, only a small portion of fishing activities are monitored (Pennino et al., 2016); however, Remote Electronic Monitoring (REM) via video cameras is a powerful and promising monitoring tool that will improve understanding of the actual impact on top predators (Course et al., 2020).
2.1.3 Visual and acoustic surveys
The abundance and distribution of top predators, such as seabirds, marine mammals, and elasmobranchs at sea can be monitored through systematic aerial and vessel surveys (e.g., Fortuna et al., 2014; Giménez et al., 2018; Louzao et al., 2019; Waggit et al., 2019) and land-based visual surveys (e.g., Arroyo et al., 2016; den Heyer et al., 2021; Gutiérrez-Muñoz et al., 2021; IJsseldijk et al., 2021). These sampling methods can produce robust absolute or relative abundance estimates (e.g., Hammond et al., 2013; Authier et al., 2018; Saavedra et al., 2018; García-Barón et al., 2019; ACCOBAMS, 2021; Hammond et al., 2021). Visual surveys may require the correction of biases associated with observers, availability of species at the surface, weather conditions, and estimation of distances in boat-based surveys (Buckland et al., 2004; Borchers et al., 2006). Under specific conditions, data collected from platforms of opportunity (e.g., from ferries: Robbins et al., 2020; cargo ships, fishing vessels: Louzao et al., 2020; or whale watching: Pérez-Jorge et al., 2016) may be used to detect relative trends and complement the knowledge, e.g., on species presence. However, the lack of a systematic data collection approach can drive biases and low predictive power (e.g., Glad et al., 2019).
Many pinniped and seabird species breed or molt in colonies where they return annually, providing a unique opportunity to record changes in the population by surveying them via land-based or aerial surveys (Russell et al., 2019; ICES, 2022). In synchronous breeders, such counts often represent either a constant and known proportion of the entire population (e.g., during seal molt; Brasseur et al., 2018) or a key subset of the population (e.g., pups or breeding pairs of seabirds). This is not the case for asynchronous breeders (e.g., grey seal Halichoerus grypus pups; Russell et al., 2019), for which colony counts often represent a slightly variable proportion of a population subset. Even though these seasonal agglomerations do not represent their distribution at sea, these counts can provide population indexes for trend assessment and demographic parameters.
Acoustic monitoring can also offer a non-invasive and cost-effective method of evaluating densities and distributions of marine predators that are difficult to observe directly in their natural habitats, such as deep-diving cetaceans, bony fish and elasmobranchs, or rare species. This technique is based on the use of hydrophones or underwater microphones to passively record vocalizations made by marine predators (e.g., Jaramillo-Legorreta et al., 2017; Amundin et al., 2022; Westell et al., 2022) or active sonars or echosounders detecting species based on their echoes (e.g., Bertrand and Josse, 2000).
2.1.4 Marking and photo-identification techniques
Top predators, such as whales, dolphins, seals, and some species of sharks that bear natural markings (e.g., dorsal fin nicks, coloration patterns) can be individually recognized through photo-identification (Hammond, 1986; Brooks et al., 2010; Pérez-Jorge et al., 2016). Seabirds and pinnipeds can be artificially marked through tags or brands (Ollason and Dunnet, 1978; Tavecchia et al., 2008; Walker et al., 2012),. Depending on the type of artificial mark, individuals may need recapture for identification (e.g., metal rings in birds) or can be “recaptured” visually. Such data can be used to estimate abundance through Mark-Recapture models (see section 3.1.2).
2.2 Indirect sampling methods to assess trends of top predator distribution and abundance
2.2.1 Biogeochemical markers to inform ecosystem modeling
Intrinsic bio-geochemical markers, such as stable isotopes, fatty acids, trace elements, and pollutant levels are commonly used in ecology to understand changes in the spatial and trophic ecology of marine top predators (Louzao et al., 2011b; Ramos and González-Solís, 2012; Kytinou et al., 2020). They can also inform on the processes behind some of the declines that marine top predators face (Jepson et al., 2016).
Over the last decades, the use of stable isotope analysis, especially those based on 13C/12C (δ13C), 15N/14N (δ15N), and 34S/32S (δ34S) ratio determinations in species tissues, has revolutionized the way we look at wild species’ trophic ecology, particularly in marine top predators (Bond and Jones, 2009; Newsome et al., 2010). These approaches provide insight into habitat use, feeding ecology, intra- and inter-specific food resource competition, migration, physiology, and nutritive condition, among others (e.g., Giménez et al., 2013, 2017; García-Vernet et al., 2021; Gaspar et al., 2022). Stable isotope ratios can also provide quantitative assessments of the multiple dimensions of the ‘ecological niche’ (Hutchinson, 1957). The term ‘isotopic niche’ was first coined by Newsome et al. (2007) and has been extensively used for addressing complex ecological questions related to intra- and inter-specific trophic interactions (e.g., Borrell et al., 2021). Recently, compound-specific stable isotopes in amino acids (CSIA-AA) have emerged as a complementary method to overcome some of the drawbacks of bulk stable isotope analysis and enhance the ability to discriminate trophic resources (Whiteman et al., 2019; Bode et al., 2022).
2.2.2 Biologging and telemetry
Animal-borne electronic devices (Ropert-Coudert and Wilson, 2005) allow the remote collection of a vast array of high-resolution quantitative data on individual distribution, movement, behavior, trophic and social interactions, and internal state (McConnell et al., 1992; Weimerskirch et al., 2012; Watanabe and Takahashi, 2013; Banks et al., 2014; Andrzejaczek et al., 2022; Papastamatiou et al., 2022; Sulikowski and Hammerschlag, 2023; Watanabe and Papastamatiou, 2023). These tools can also be used to estimate at-sea species distributions (e.g., Aarts et al., 2008; Louzao et al., 2011c; Carter et al., 2022). The data can be stored (in archival devices) or sent remotely (through ARGOS, VHF/UHF, or GSM). The most common types of data collected are position (through geolocation, ARGOS, or GPS), acoustic, diving, and speed data. Ancillary environmental data (e.g., temperature) can also be collected (Charrassin et al., 2008). The multi-parametric sensors in these devices allow the physical characterization of the environment, effectively turning animals into ‘biological samplers’ (McMahon et al., 2021; Holland et al., 2022). These data can also help estimate mortality rates (Heupel and Simpfendorfer, 2002) and define populations (Lewis et al., 2009). Although they do not allow the estimation of abundance indexes, they are essential for improving abundance estimates obtained through other methods, for example by providing species information on time spent at the surface (i.e., availability bias in Distance Sampling) in relation to specific physiographic and behavioral conditions (e.g., Louzao et al., 2011c; Hagihara et al., 2016).
2.2.3 Environmental DNA
The environmental DNA (eDNA, i.e., the genetic material released to the environment by organisms) is an emergent, powerful approach to marine top predator monitoring (Foote et al., 2012; Albonetti et al., 2023; Jenrette et al., 2023). DNA traces of top predators can be retrieved from the environment by filtering water to confirm species’ presence in areas where they were not visually detected (Postaire et al., 2020). The metabarcoding allows the simultaneous identification of several taxa using short, conserved DNA fragments (primers), amplifying the DNA of the taxa of interest (e.g., Bakker et al., 2017). The species-specific assays target single (or a few) species and can be used to detect the presence of top predators (e.g., Budd et al., 2021). Both approaches can potentially contribute to megafauna monitoring (Suarez-Bregua et al., 2022), particularly for the most elusive or rare species (e.g., Juhel et al., 2021; Faure et al., 2023), such as deep-diving odontocetes or sharks. Recent studies have successfully used DNA metabarcoding to describe top predators’ diets using stomach contents or fecal samples (de Sousa et al., 2019).
2.2.4 Remote sensing and other digital tools
Remote sensing technologies also provide a non-invasive means for evaluating top predators’ presence, distribution, and behavior. For instance, satellite-based monitoring can help determine the presence and distribution of marine mammals, elasmobranchs, and seabirds in vast areas (e.g., McConnell et al., 1992; Fretwell et al., 2014; Labrousse et al., 2022). Unmanned vehicles, such as drones, autonomous underwater vehicles (AUVs), and remotely operated vehicles (ROVs) equipped with cameras, acoustic sensors, and other instruments, can also be used to collect data on the size, distribution, and behavior of marine predators (e.g., Giacomo et al., 2021). This information can also be obtained from baited fixed cameras deployed in inaccessible areas where top predators aggregate or individuals are attracted (e.g., Currey-Randall et al., 2020).
Monitoring of top predators can benefit from ongoing social digitalization and emerging disciplines such as culturomics and iEcology (Jarić et al., 2020). From one side, hyper-connectivity through social media and digital platforms can boost citizen/community science programs by increasing engagement and participation. On the other hand, passive mining of the digital activity of users can complement traditional methods in tracking the occurrence of top predators (Morais et al., 2021; Sbragaglia et al., 2024). The main advantages of emerging digital monitoring are reduced data collection costs and almost real-time data (Lennox et al., 2022). Disadvantages are reduced data quality and reliability (e.g., data tend to be biased by the lack of research design that affects, for example, temporal and spatial coverage) and limited usability (e.g., such data may be valuable for ‘presence’ only studies on top-predators only if the issue of ‘lack of reporting species absence’ is considered and temporal and spatial observation coverage is large); these limits imply major investments on data quality and data mining (Aceves-Bueno et al., 2017).
3 Modeling approaches to detect trends of marine top predators
The monitoring approaches previously discussed provide data on the abundance and distribution trends of marine top predators that need to be analyzed. Both data-driven models and models based on first-principle assumptions and biological mechanisms can directly use monitoring data. In this section, we review both dimensions and categorize modeling techniques according to their main targets (species, community, and ecosystem).
3.1 Population and demographic parameters and models
3.1.1 Distance sampling
The most common methodology to estimate the abundance and distribution of top predator species at sea is Distance Sampling (Buckland et al., 2004). This statistical method calculates distances to the animals (e.g., seabirds and marine mammals) from predefined line-transects or fixed positions. The method estimates the detection probability function based on the sampled distances between the observer and the animals/groups (Buckland et al., 2004). This methodology has been successfully used to estimate the large-scale abundance of cetaceans, elasmobranchs, and sea turtles and detect trends (e.g., Hammond et al., 2013; Fortuna et al., 2014; Authier et al., 2018; Hammond et al., 2021).
3.1.2 Mark-recapture methods
Recaptures of previously marked individuals allow monitoring the absolute marine top predator abundance throughout mark-recapture estimators (Barbraud and Weimerskirch, 2003; Cooch and White, 2008; Hammond, 2010), which can also be used to detect changes on demographic parameters (e.g., birth, survival/mortality, emigration/immigration rates, growth rates; Genovart et al., 2016; Lunn et al., 2016; Verborgh et al., 2019).
3.1.3 Stock/population assessments
Population models are frequently used in stock assessments to inform Regional Fisheries Management Organizations, such as the International Commission for the Conservation of Atlantic Tunas (ICCAT), the International Council for the Exploration of the Sea (ICES), and the General Fisheries Commission for the Mediterranean (GFCM). The age-structured stochastic modeling approach, used to assess Atlantic bluefin tuna dynamics and to predict the future development of fish populations (over 10-20 years) under different fishing mortality and population biology scenarios (e.g., growth rates, maturity schedules, reproduction rate; MacKenzie et al., 2009, 2021), informed the recovery plan for this species. Population models integrate empirically derived estimates of the uncertainty of input variables to estimate probabilistic outputs of population variables (e.g., biomasses) and information on biological and fishing mortality rates from assessments.
The International Whaling Commission (IWC) conducts assessments of cetacean populations rather than of species, which is the IUCN approach. This is because local populations within a species may face very different conditions and threats, and some may be thriving, whereas others may be at risk of geographical extinction. The IWC assessments, mostly done for baleen whale populations, are based on a Bayesian logistic population dynamics model (Punt and Donovan, 2007), which incorporates information on current and pre-exploitation absolute abundance estimates, a species-specific productivity parameter, time-series of human-induced mortality (catch and bycatch), and factors to account for environmental variability. The Bayesian approach allows the downweighting of noisy input data (IWC, 1999).
The IUCN species assessments are most commonly semi-quantitative, allowing inferred trends to be based on expert knowledge and semi-quantitative data. However, there is an option for “quantitative analysis” (i.e., criterion E), which includes the Population Viability Analysis (PVA). A PVA is a model investigating how several known factors interact and determine the risk of extinction for a population, given a set of conditions, including a certain timeframe. Criterion E is seldom used for marine top predators as it requires background knowledge of ecological, genetic, and demographic parameters (including spatial distributions of suitable habitat, patterns of occupancy, and habitat relationships) that are usually unavailable. Nevertheless, for certain marine predator populations, PVAs are possible (e.g., Balearic shearwaters, Puffinus mauretanicus; Oro et al., 2004; California sea lions, Zalophus californianus; Hernández-Camacho et al., 2015). The IUCN Green Status of Species (Box 3) is a complementary tool to the Red List, which assesses the recovery and conservation success of species. A species is considered “fully restored” if it meets three conditions throughout its range (including historical areas): it is present, is not threatened with extinction, and performs its ecological functions.
Box 3. - The IUCN Green Status tool: putting the Red Listing into a historical perspective
The IUCN Red List of Endangered Species is a globally recognized benchmark for assessing the threat of extinction that certain animal, fungus, and plant species face. The IUCN Green Status of Species is a relatively recent and complementary tool (available since 2020) that assesses the recovery of species populations and measures their conservation success. A species qualifies as “fully recovered” if, in all parts of its range (including those occupied historically), it satisfies three conditions: it is present (i), is not threatened with extinction (ii), and performs its ecological functions (Akçakaya et al., 2018).
Of the seven most commonly feared top predators listed in the Introduction, only for the white shark (Carcharodon carcharias) the IUCN has produced a global and regional (i.e., Mediterranean Sea and Europe) Red List assessment (‘Vulnerable’ and ‘Critically Endangered’, respectively) and a Green Status is “Moderately Depleted”. At present [on 15/06/2023], the IUCN Green Status has been given to 37 animal species. Of these, only 10 are linked to the marine environment, and only one has been assessed as ‘Fully Recovered’, the banded wobbegong in Australia. It is worth noting that being classified as ‘Least Concern’ does not mean being ‘Fully Recovered’, with the Eurasian otter being an extreme case of a LC species still considered ‘Largely Depleted’. This highlights the importance of the historical context.
For the other six most commonly feared top predators mentioned in section 1.1, only Red List assessments are available. The sand tiger shark is assessed as Critically Endangered at global and regional levels with a decreasing trend. The Polar bear is assessed as Vulnerable (Wiig et al., 2015), with an unknown global trend and a decreasing trend in Europe (Wiig et al., 2007). The same applies to the bull shark with a global decreasing trend. Sperm whales, which suffered overexploitation by the whaling industry until the late 1980s and extremely high mortality due to bycatch in large driftnets until the early 2000s, are currently assessed as Vulnerable at the global scale, but Endangered in the Mediterranean Sea, with a decreasing trend. Leopard seals are classified globally as Least Concern. The Killer whale is assessed as Data Deficient. The lack of Green Status for these and other top predator species limits the ability of managers to fully understand the extent and the meaning of their declines and the level of concern around their regional and global conservation status. Green Status assessments should be systematized and realized in synergy with Red List Assessments.
3.2 Species distribution models
Species distribution models (SDMs) can be used to predict the spatial presence and distribution of marine species based on their relationship with environmental variables (Guisan and Zimmermann, 2000). They can fit to presence/absence, density, or presence-only data (e.g., generalized linear or additive regression models, classification and regression trees, autoregression models). This modeling approach can be seen as an operational application of the ecological niche (Hirzel and Le Lay, 2008). SDMs are also used to predict species distribution under varying climate change scenarios (e.g., Russell et al., 2015; Moullec et al., 2022). Ensemble SDMs have been used to predict changes in marine species distribution (Lotze et al., 2019; Tittensor et al., 2021; Erauskin-Extramiana et al., 2023). SDMs accounting for the potential distribution prediction uncertainty and for relationships with key environmental variables on a regional or global scale can be used to inform mechanistic ecosystem models (Coll et al., 2019a).
SDMs have been widely used to predict distributions and identify geographical regions suitable for different cetacean species (e.g., Fortuna et al., 2018; Giménez et al., 2018; Chavez-Rosales et al., 2019; García-Barón et al., 2019; Ramírez-León et al., 2021), seabirds (e.g., Louzao et al., 2006; Oppel et al., 2012; Frederiksen et al., 2013; Astarloa et al., 2021), elasmobranchs (e.g., Pennino et al., 2013; Lauria et al., 2015; Follesa et al., 2019; González-Andrés et al., 2021), pinnipeds (Aarts et al., 2008) and combined taxonomic groups (e.g., Louzao et al., 2019; García-Barón et al., 2020).
3.3 Ecosystem modeling: from energy flows to multispecies and food-web interactions
3.3.1 Stable Isotope mixing models and trophic position
Stable isotope analyses have emerged as a suitable alternative to conventional approaches to reconstruct the individuals’ and populations’ assimilated diet and trophic position through mass-balance mixing models (e.g., Navarro et al., 2009; Gaspar et al., 2022). Bayesian statistics allow adding priors to modeling diet mixtures. They also allow adding fixed and random effects as covariates explaining variability in mixture proportions and calculating relative model support through information criteria (Stock et al., 2018; Lloret-Lloret et al., 2020).
Trophic Position (TP) is commonly used to describe the trophic structure and relationships at the community level and to study the effects of human and environmental changes on marine food webs. In trophic studies, when δ15N baseline and predator values are known, the use of this isotope is common practice to calculate the TP. Additionally, the use of compound-specific stable isotopes in amino acids (CSIA-AA) has recently enabled modeling TP using only values from the predator, as some amino acids are considered source (i.e., baseline) and others trophic (Bode et al., 2022).
3.3.2 Bioenergetic models
Bioenergetics modeling provides a mechanistic basis for projecting climate change effects on marine living resources. It has been applied widely to fish, marine mammals, and other taxa (Rosen and Trites, 2000; Winship et al., 2002; Fortune et al., 2013; Rechsteiner et al., 2013; Louzao et al., 2014; Jeanniard-du-Dot et al., 2017; Booth et al., 2023). These approaches are often species-specific, and integrating data related to individual and short-term processes into population dynamics can be challenging. Additionally, major challenges arise from climate change projections centered on predictions of organism and population responses to novel environmental conditions, which may strain current modeling capabilities (Moullec et al., 2022; Erauskin-Extramiana et al., 2023).
3.3.3 Multispecies models
Several statistical and mechanistic approaches exist to simultaneously model multiple species. For example, Joint Species Distribution Models (JSDM) integrate species interactions into metacommunity and macroecology (Tikhonov et al., 2020). JSDM allows for integrating data on species densities, environmental covariates, species traits, phylogenetic relationships, and spatio-temporal information. This approach enables the analysis of species occurrence patterns, which can be decomposed into environmental responses and residual correlations not explained by predictors (Hui, 2016), potentially indicating biotic interactions. In a recent JSDM application in the Bay of Biscay, Astarloa et al. (2019) demonstrated that the co-occurrence patterns of top predators (marine mammals and seabirds) and prey (pelagic fish and crustaceans) were driven by a combination of environmental and biotic factors. Many multispecies mechanistic models exist (Plagányi, 2007), including models of intermediate complexity (Plagányi et al., 2012). Additionally, empirical relationships of biomass and abundance estimates obtained from observations and population models have been used to establish links between predator requirements and prey. For example, one study links seabird colony-years per breeding site to the abundance of principal prey for each species, determining the proportion of prey abundance needed to ensure seabird success (Cury et al., 2011).
3.3.4 Marine ecosystem models (EwE, SNS, Mizer)
Ecological processes and human activities can be explicitly incorporated into process-based marine ecosystem modeling (Fulton, 2010; Peck et al., 2018; Tittensor et al., 2018; Moullec et al., 2022), as in Ecopath with Ecosim and Ecospace models (EwE hereafter; Christensen and Walters, 2004). These tools allow for building food-web models by describing the ecosystem as energy flows between functional groups, each representing a species, a subgroup of a species (e.g., juveniles and adults), or a group of species with functional and ecological similarities. Ecospace is the spatial-temporal dynamic module of EwE, allowing temporal and spatial 2D dynamics representation of trophic web components.
EwE has been widely applied to analyze the spatial impacts of fisheries, management scenarios (e.g., marine protected areas, MPAs), and climate change on marine species and ecosystems. This is achieved by linking Ecospace with low trophic level models (Fulton, 2011) or external spatial-temporal data (Steenbeek et al., 2013) and developing spatial optimization routines (Christensen et al., 2009). An addition to the spatial-temporal modeling capabilities of EwE is the Habitat Foraging Capacity model (Christensen et al., 2014). This model allows for the spatial derivation of foraging species’ capacity from cumulative effects of multiple physical, oceanographic, environmental, and topographic conditions in conjunction with the food web and fisheries dynamics. This integration bridges the gap between envelope environmental and food-web models (Coll et al., 2019a). EwE has been used to assess the role and dynamics of predators in marine ecosystems, such as sea otters (Espiro et al., 2011), endemic skates (Coll et al., 2013), tunas (Cox et al., 2002), and Steller sea lions (Guénette et al., 2006). It is increasingly used to assess the effect of cumulative impacts in the ocean (de Mutsert et al., 2023), including underwater noise (Serpetti et al., 2021), and to study global scale dynamics through hybrid modeling approaches (Coll et al., 2020).
4 Historical perspective and ecological implications
Understanding the ecological status of top predator populations is essential to identify the key measures required for their effective conservation. These species are ecosystem sentinels that respond to ecological fluctuations of ecosystems and generate essential information about the ecological implications of other organisms (Hazen et al., 2019). The long-term historical exploitation of large predators has influenced their contemporary abundance. Thus, neglecting historical data may lead to excessively optimistic assessments of their conservation status, lower recovery targets, and larger exploitation quotas than if the historical perspective is considered (McClenachan et al., 2012). Shifting baselines (Pauly, 1995) can result from the intergenerational loss of knowledge regarding species abundance, directly affecting how species and ecosystems are perceived and managed. Historical data allow scientists and managers to understand species and population dynamics better and make informed decisions promoting the long-term sustainability of marine populations.
Notwithstanding the lack of reliable data from the pre-industrial fishing age (ca. 1960 and back), global oceans are estimated to have lost 90% of the biomass of large predatory fish species since the start of industrialized fisheries, with major stock biomass declines of up to 80% within 15 years of industrialized exploitation (Myers and Worm, 2003). Paleczny et al. (2015) conducted a global meta-analysis and reported that seabird populations declined by an average of 69% from 1950 to 2010. Certain groups, such as albatrosses and petrels, experienced even more pronounced declines. McCauley et al. (2015) reported a decline in marine mammal and seabird populations worldwide by 45% and 28%, respectively, over the past 40 years.
Incorporating historical data into assessments of marine populations frequently reveals more severe declines that may go unnoticed when relying solely on short-term observations. A meta-analysis of instantaneous rates of change for blue sharks (Prionace glauca) in the Mediterranean indicated population declines of 97%. This suggests a baseline population size 2.5 times higher than that derived from earlier estimates based on comparisons of CPUEs between 1978 and 1999. This conclusion was based on a comprehensive data series beginning in 1950, including commercial landings, scientific surveys, and sighting records (Ferretti et al., 2008).
A large-bodied fish whose population collapsed before standardized monitoring began during the 1950s is the critically endangered common skate (Dipturus batis). Bom et al. (2022) placed the recent increase in population numbers in the North Sea in a 120-year perspective by examining various recent and historical data of standardized capture counts. The species had a relatively high abundance between 1901 and 1920, followed by a steady decline from 1920 onwards, nearly leading to extinction around 1970 in the North Sea. The authors found that the current abundance of the species is still well below historical baselines and shows a slight recovery only at the far north edge of its geographical range.
A long-term perspective is crucial to avoid overly optimistic assessments, even for recovering populations. The standardized sampling of marine populations began in the 1970s or later in most regions, after many species had already experienced significant declines or collapse. This can lead to overstating recent recovery levels of top predator populations (Bom et al., 2022). For instance, the southern right whale (Eubalena australis) has experienced centuries of exploitation. The pre-exploitation abundance in the southwestern Atlantic Ocean was estimated at roughly 58,000 individuals, and it dropped to its lowest levels in the 1830s, with fewer than 2,000 individuals remaining. The current median population estimate is about 4,700 whales, indicating a certain recovery but much lower numbers than the pre-exploitation period (Romero et al., 2022).
Setting realistic goals for conservation efforts requires comprehensive knowledge of abundance over an ecologically meaningful “long time” period. An emblematic example is the large Gulf grouper (Mycteroperca jordani) in the Gulf of California (Saénz-Arroyo et al., 2005). Based on increased catch from data systematically collected since 1986, an annual catch increase of up to 5% was recommended in 2000. However, integrating historical evidence, observations from naturalists, and systematic documentation on fishers’ perception of the abundance of this species, revealed that the Gulf grouper had undergone an alarming decline since the peak of the Gulf grouper fishery before the 1970s. It is worth noting that this decline occurred well before formal fishery statistics were established.
Long-term time series may provide data supporting a more robust understanding of the potential future trajectories of change in population distribution and abundance, for example, in response to climate change. We currently have limited knowledge of the climate change-induced processes that shift the distribution of top predators, particularly in amplitude and lagged processes (Lan et al., 2021). Louzao et al. (2013) showed a progressive habitat shift, between 1958 and 2001, for the Wandering Albatross (Diomedea exulans) of recurrent, occasional, and unfavorable foraging habitats, driven by the propagation of sea surface height from SE South Africa towards Antarctica. Using relatively long-term time series data (1988-2018) from two fjords in West Spitsbergen (Svalbard), Descamps and Ramírez (2021) investigated the relationship between sea ice extent and population size of two of the most prevalent Arctic seabirds, the Brünnich’s guillemot (Uria lomvia) and black-legged kittiwake (Rissa tridactyla). The authors concluded that the ongoing decline in Arctic Sea ice plays a role in Arctic seabird population trajectories, even if its disappearance on the breeding grounds is likely not the main driver of change in seabird populations.
Historical data have a high potential for application in “data-poor” stock assessments, where reference points and recovery targets are often established using a variety of data types, limited in quality, quantity, and coverage. One example of a marine top predator stock assessment based on historical data is the case of the Northwest Atlantic population of the white shark (Curtis et al., 2014). In the early 20th century, white sharks were commonly caught as bycatch in commercial fisheries targeting other species, such as tunas and swordfish. The authors used historical data from various sources, including newspaper articles, fishery records, and interviews with fishers and other experts to understand the past trends and current status of the white shark population. This information, combined with recent data from tagging studies and aerial surveys, indicated that the Northwest Atlantic population of white sharks had declined by approximately 73% (median estimate) between the mid-1970s and throughout the 1980s. The white shark relative abundance stabilized during the 1990s then increased during the 2000s until the end of the study (i.e., 2010). The increase was linked to the implementation of specific fishery management measures, including species protection.
More prominently, historical data are key in extinction risk assessments such as those coordinated by the IUCN Red List, which estimates population changes over ‘10 years or three generations of a species, whichever is the longer’. Given the inherent generation length of top predators, these assessments are frequently hindered by a lack of data, particularly for marine mammals, elasmobranchs, large teleosts, and seabirds, which in many cases are long-living species. Ascension Island has the largest colony of sooty terns (Onychoprion fuscatus) in the Atlantic Ocean, and censuses between 1990 and 2013 have shown that its population size is static. However, historical data showed that the breeding population contained over 2 million individuals in the 1870s and remained at this level for at least 70 years. The population declined from > 2 million in 1942 to 350,000 birds by 1990. The population trend spanning a period equivalent to three generations of the species (63 years; 1942–2005) showed an approximate 84% decline (Hughes et al., 2017). Using IUCN criteria, sooty terns on Ascension could be considered ‘Critically Endangered’; hence Hughes et al. (2017) concluded that re-evaluating its conservation status is necessary at the local level and possibly globally.
Seals have been severely exploited for centuries, primarily for oil rather than fur, which became a later cause for their demise. In Western Europe, for example, grey seals (Halichoerus grypus) were numerous based on archaeological findings (Reijnders et al., 1995) but completely disappeared from the continental coasts before the Middle Ages. After protection in the United Kingdom at the beginning of the 20th century, grey seal populations gradually recovered and re-colonized most of their former distribution (Brasseur et al., 2015). Estimates of former population sizes of severely hunted species can be back calculated from well-documented hunting records. For example, annual catch data were used to estimate the potential size of the harbor seal population in 1900 (Reijnders, 1992). However, bounties and regular hunting in previous centuries had already decreased the population by 1900 (de Vooys et al., 2012). This is an example of shifting baselines and highlights the need to put things into perspective also when reconstructing the sizes of top predator populations from historical data.
4.1 The human factor in marine top predators decline
Humans are playing a main role on marine top predators’ decline. This ranges from being the cause of direct mortalities - either purposefully (i.e., fishing and hunting) or accidentally (i.e. fishery bycatch and collisions with ships) - to causing the widespread and, at times subtle, habitat degradation (e.g., food web disruption, habitat loss and fragmentation, seafloor damage, coastal zone modification, chemical and acoustic pollution, climate crisis, etc.).
The depletion of populations due to overfishing or overhunting has been identified or suspected as a major cause of the decline for many marine top predators (Pauly et al., 1998; Stevens et al., 2000; Myers and Worm, 2003; Lotze and Worm, 2009). A recent review of marine extinctions (Nikolaou and Katsanevakis, 2023) reported 8 cases of top predators’ global extinctions (4 seabirds, 3 marine mammals, and 1 teleost fish) and 89 cases of local extinctions; the main driver of extinction of top predators was human-induced direct mortality (i.e., overexploitation and bycatch).
Bottom-up processes related to the overexploitation of lower trophic levels cause a reduction in food for higher-trophic level animals such as seabirds and marine mammals, potentially resulting in losses in reproduction or reductions in their population size (Myers et al., 2007; Terborgh and Estes, 2013).
The harmful consequences of the exposure of individuals to certain pollutants are also recognized as a primary driver of the decline of top predators. Most pollutants tend to accumulate (bioaccumulation) in marine organisms and are eventually transferred along the food web (biomagnification) with significant consequences for top predators (Kelly et al., 2009). Top predators are, therefore, under pressure from pollution and can also serve as sentinel species for monitoring the environmental health of the marine environment they inhabit (Garcia-Garin et al., 2021; Garcia-Garin et al., 2022).
In addition, given that climate change is expected to have a major impact on marine top predator species’ distributions and abundances, marine conservation and management efforts will need to consider these ongoing changes and factor them in their decision-making (e.g., Braun et al., 2023).
5 Examples of assessments linked to policy frameworks
This review does not have a specific focus on the EU Habitats Directive, as this has been absorbed into the conservation and management approaches of the more recent MSFD and MSP directives. However, both sections 5.1 and 5.2 refer to several aspects and tools deriving from the Habitats Directive (e.g., links to HD monitoring and assessment framework in Commission Decision 2017/848; Natura 2000 network, etc.).
5.1 Assessment examples
The EU MSFD and UN Regional Sea Conventions aim to improve the governance of the marine regions surrounding the European continent and reinforce the protection of the marine environment through cooperation among all riparian countries. The MSFD aimed to achieve or maintain GES for European seas by 2020. Top predators are considered in the MSFD assessments under three descriptors: D1 ‘Biodiversity’, D3 ‘Fishing’, and D4 ‘Food webs’. Under D1, MSs consider 139 species of birds, 40 species of marine mammals, and 321 species of fish. The latter includes elasmobranchs and commercial species that may be assessed under D3 and D4 (EC, 2018; JRC, 2018).
A recent review of the MSFD reports (for the reporting cycle 2012/13-2018) of a sample of nine Member States (Croatia, Estonia, Finland, France, Germany, Malta, Netherlands, Romania, and Spain; Franco et al., 2021) has shown that, amongst the bird species most commonly assessed under the MSFD D1, there are terns (little tern Sternula albifrons, common tern Sterna hirundo, and Sandwich tern Sterna sandvicensis) in the Baltic, Atlantic, Mediterranean, and Macaronesia, cormorants (European shag Phalacrocorax aristotelis and great cormorant P. carbo) in the Atlantic, Baltic, and Mediterranean, and Scopoli’s shearwater (Calonectris diomedea) in the Mediterranean Sea. The assessments focused, in particular, on their breeding colonies. Small-toothed cetaceans, such as the common bottlenose dolphin (Tursiops truncatus) and harbor porpoises (Phocoena phocoena), and grey seals are amongst the most frequently reported sea mammals, depending on the regions, with the bottlenose dolphin and grey seal being most often reported as in ‘Good status’. In contrast, harbor porpoise is often classified as ‘Not-good status’ (Franco et al., 2021). As for predator fish, commercial species including gadoids (e.g., Gadus morhua, Micromesistius poutassou), seabass (Dicentrarchus labrax), bluefin tuna and turbot (Scophthalmus maximus), as well as elasmobranchs such as rays (e.g., Raja clavata) and dogfishes (e.g., Scyliorhinus canicula, Squalus acanthias), are the most commonly reported fish under D1, D3 or D4.
The status of individual species (‘Element status’ in MSFD reports) is the integration of the status assessment of a set of criteria based on established indicators. Examples of the D1 criteria and associated indicators used by Member States to assess the state of seabirds and mammals are given in Table 1, with a reference to the homologous indicator used by or adopted from RSCs. The EU European Information System WISE-Marine provides a useful comparative table on the European and Regional Indicators used in the GES assessment by various RSCs. Table 1 shows only the ‘state’ criteria, not ‘pressure’; hence fishery-related mortality (D1C1) was excluded.
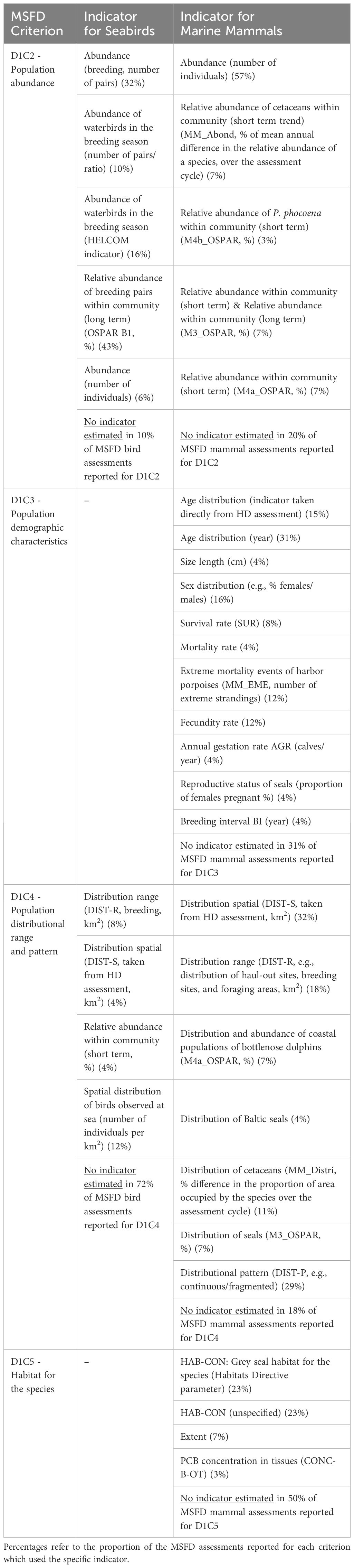
Table 1 MSFD D1 criteria and indicators for seabirds and marine mammals (see Commission Decision (EU) 2017/848), with reference to OSPAR and HELCOM analogous indicators, as reported by a sample of nine Member States in 2018 MSFD reports (source: Franco et al., 2021).
Most of the indicators used for MSFD D1 assessments align with those used in assessments by RSCs. The MSFD Art 5(2) and the more recent Commission Decision (EU) 2017/848 explicitly require Member States to ensure that the implementation of the different articles is coherent and coordinated across the region or subregion. From a geographical perspective, the lowest level of harmonization (in terms of indicator re-use) occurs in the Barcelona Convention region, whereas the highest level of re-use was observed for the Netherlands, followed by France and Germany (Franco et al., 2021). The highest level of harmonization between MSFD and RSCs appears to occur for marine mammals (compared to marine reptiles, birds, and benthic habitats), as suggested by the re-use of assessments, from the monitoring data to the indicators used (Franco et al., 2021). This is likely the result of the RSCs having established methods for marine Great cormorant, mammal data collection as well as other international agreements, such as ACCOBAMS (the Agreement on the Conservation of Cetaceans of the Black Sea, Mediterranean Sea, and contiguous Atlantic area) and ASCOBANS (the Agreement on the Conservation of Small Cetaceans of the Baltic and North Seas), which have promoted common standards and established data flows. Harmonizing the Barcelona Convention’s Ecosystem Approach (Barcelona Convention 2008, 2012, 2013, 2016) with the MSFD is ongoing; major improvements and a quasi-complete alignment are expected in the next triennium.
Franco et al. (2021) showed that population abundance (D1C2) for birds and population abundance (D1C2) and distributional range and pattern (D1C4) for mammals were the criteria most successfully assessed by Member States, i.e., sufficient data and established indicators for these allowed the status to be classified as ‘good’ or ‘not good’ in most cases.
Although Franco et al. (2021) did not consider it in their evaluation, the criterion D1C1 is fundamental to assessing the biodiversity GES. D1C1 quantifies the ‘mortality rate per species from incidental bycatch’ and prescribes that fishery-induced mortality is kept ‘below levels which threaten the species, such that its long-term viability is ensured’ (Commission Decision (EU) 2017/848). In terms of policies, this criterion is linked to the concept of EBM and various targets of the EU Common Fisheries Policy on reducing bycatch and discards. The species concerned are potentially all ‘non-commercially-exploited species (incidental bycatches)’. Despite the recent EC Communication on the EU Action Plan on ‘Protecting and restoring marine ecosystems for sustainable and resilient fisheries’ (EC, 2023), which calls for concrete actions on D1C1 by EU Member States by the end of 2023, nothing is ready to be adopted. In particular, no major improvements are seen regarding officially adopting threshold algorithms to estimate the ‘maximum allowable mortality rate from incidental catches’, nor a fully operational monitoring system is in place for the EU fleet to gather appropriate data on bycatch rates (ICES, 2021). Moreover, both EU and national fishery management frameworks are not adequately prepared to: (i) use such thresholds to assess their sustainability, ensuring the long-term viability of concerned species; and (ii) minimize the effect of recorded bycatch rates to enable the full recovery of concerned species and populations. The ultimate deadline to realize and implement such frameworks for all species is 2030.
5.2 Area-based tools to implement an ecosystem approach
The current trajectories of changes in top predators and the complexity of monitoring and understanding the factors affecting their long-term viability call for a holistic approach to their conservation and management. A key management tool to conserve their habitats is MPAs. MPAs have proven to be effective in conserving and restoring ecosystems and marine species (Leenhardt et al., 2015; Giakoumi et al., 2017; Pérez-Roda et al., 2017), and protecting important marine habitats for top predators (Gormley et al., 2012). However, MPAs are often too small or inappropriately designed to be effective for the conservation of wide-ranging top predators, also considering the level of pressure and degradation of the unprotected surrounding ecosystems (Fortuna et al., 2018). To fully harness MPAs strengths, their designation should be incorporated into Maritime Spatial Planning (MSP). MSP is an adaptive EBM tool aiming to define the spatial allocation of human activities at sea. MSP addresses emerging challenges resulting from increasing human activities and their impacts on threatened marine ecosystems, aiming to manage oceans sustainably (Gissi et al., 2019). Guidelines to assist in the definition of appropriate time and space requirements to better meet fisheries management objectives within an MSP context have been also proposed (Dunn et al., 2011). MSP should be adopted also for the designation of Other Effective area-based Conservation Measures (OECMs) representing a novel conservation approach distinct from MPAs, contributing to conservation goals as a byproduct of other management objectives on specific human activities (Laffoley et al., 2017). However, human-wildlife interactions are still scarcely explicitly addressed in planning and rarely in MSP, and should be further implemented given the evidence of positive results (Shabtay et al., 2020; García-Barón et al., 2021).
Recently, structured public consultation involving stakeholders has demonstrated that MSP can address shark attack risk while considering multiple sea uses and conservation objectives. This highlighted the importance of integrating shark risk as a driver in the MSP process and developing a transparent, sustainable, and evidence-based public policy for managing shark risk within a broader social-ecological spectrum of stakes (Shabtay et al., 2020).
It must be stressed that the designation of EU MPA networks (Natura 2000) within MSP has often lacked systematic conservation planning principles, such as connectivity, adequacy, representativeness, and efficiency, and its focus has been more on structural characteristics of habitats and iconic species and populations in unfavorable conservation status rather than on ecosystem functioning and whole biodiversity (Katsanevakis et al., 2020). Robust and systematic approaches are necessary to recover predators and prey with threatened status. In this context, systematic conservation planning tools such as Marxan, the open-source R Prioritzr or Zonation are useful for finding a solid planning scenario that balances conservation and socio-economic perspectives (Afán et al., 2018; Giménez et al., 2020; García-Barón et al., 2021). Other spatial analyses based on GIS have been developed to incorporate the complexity of spatial management (Queirós et al., 2016), including the identification of specific areas for the protection of species at risk (Louzao et al., 2006, 2012; Coll et al., 2015). Mechanistic models (such as Ecospace) can assess the effects of management on marine ecosystems, including top predators, while considering the impacts of climate change and human activities in the ocean (Fulton et al., 2015; Gomei et al., 2021). Better systematic conservation planning accounting for functional connectivity and climate change impacts is recommended to improve the status of this key biodiversity component (Katsanevakis et al., 2020).
6 A systematic global review on success stories: factors for success
A systematic global review was conducted to identify success stories in managing threats to top predator populations, applying the PRISMA-EcoEvo approach (Preferred Reporting Items for Systematic Reviews and Meta-Analysis extension for Ecology and Evolutionary Biology; Moher et al., 2009; O’Dea et al., 2021). Details on the methods used and additional results are shown in Supplementary Materials. The aim was to identify (a) the concerned threats, (b) the types of conservation actions applied, (c) how their performance was assessed, (d) the factors contributing to their success or failure, and (e) the stakeholders involved in these success stories.
Studies included in the review met two criteria: (1) one or more populations of a marine top predator were assessed, and (2) one or more successful conservation actions were described (i.e., actions that led to a population increase/recovery or status improvement or were successful in mitigating specific threats in pilot trials). In total, 481 success stories were identified, and 181 studies were included in the review (Supplementary Figure 1; Supplementary Material). The extracted data from the reviewed papers were classified into five categories: (1) bibliographic information; (2) species-specific and study-specific information; (3) information on conservation actions; (4) participation of stakeholder groups; and (5) threat(s) mitigated through the conservation action(s). Complementary data were extracted from the IUCN Red List (IUCN, 2023) (i.e., the IUCN status and trend of the assessed species and populations).
Most success stories referred to seabirds (53%), followed by marine mammals (24%), elasmobranchs (12%), and large teleosts (11%) (Figure 1A). Over 50% of these success stories occurred in temperate regions of South America, Southern Africa, and the Northern Atlantic (Figures 1B, 2). The country with the most reported success stories was South Africa (18%), followed by Australia (14%), the US (13%), and Brazil (11%) (Supplementary Figure 6). However, the global distribution of success stories varied by taxon (Supplementary Figures 2–5).
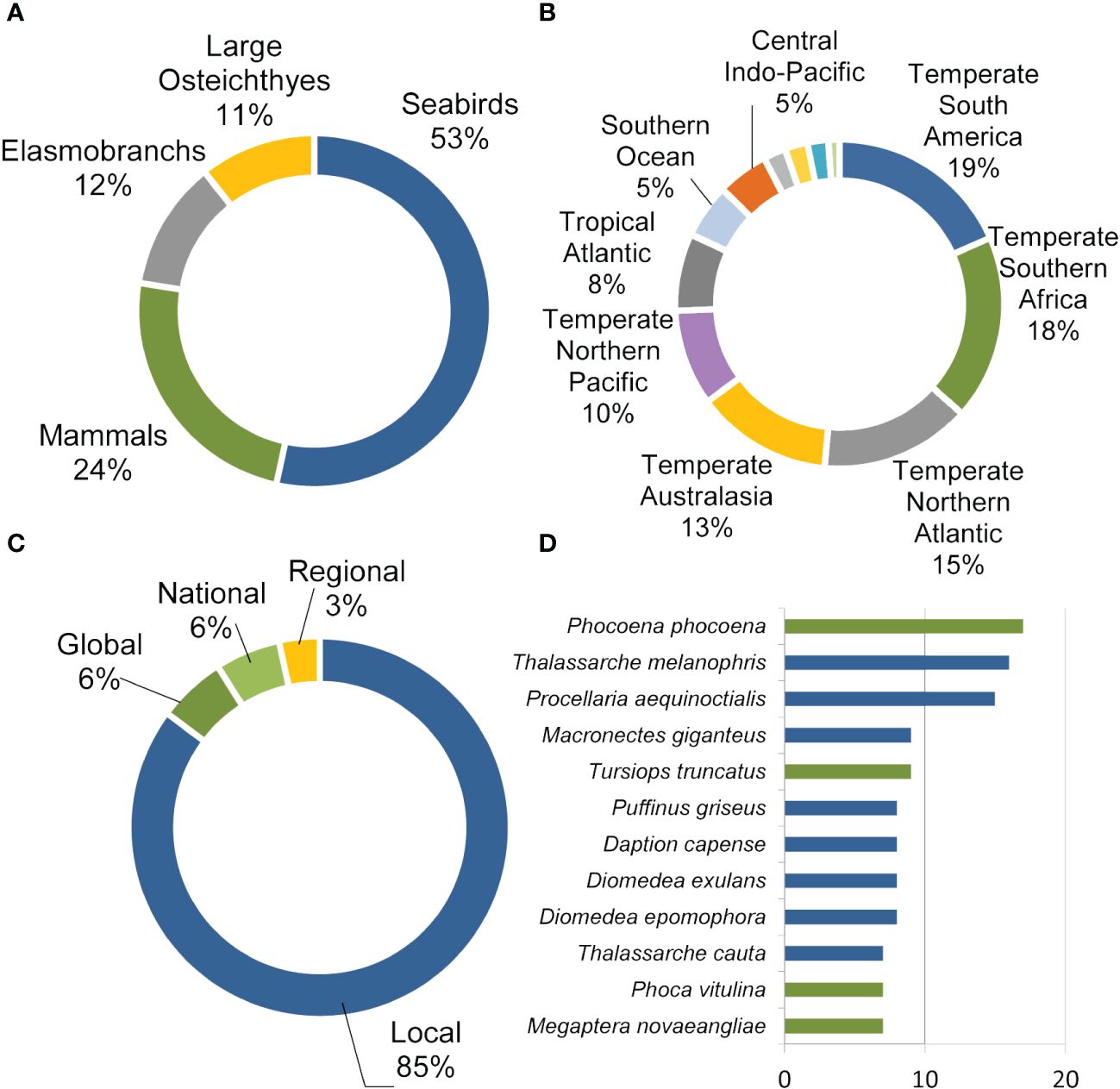
Figure 1 (A) Share of the four taxonomic groups in the success stories retrieved through the systematic review. (B) Distribution of success stories in marine realms (sensu Spalding et al., 2007). (C) The scale of management measures implemented in success stories. (D) Most commonly targeted species by management measures in the reviewed success stories (green: mammals, blue: seabirds).
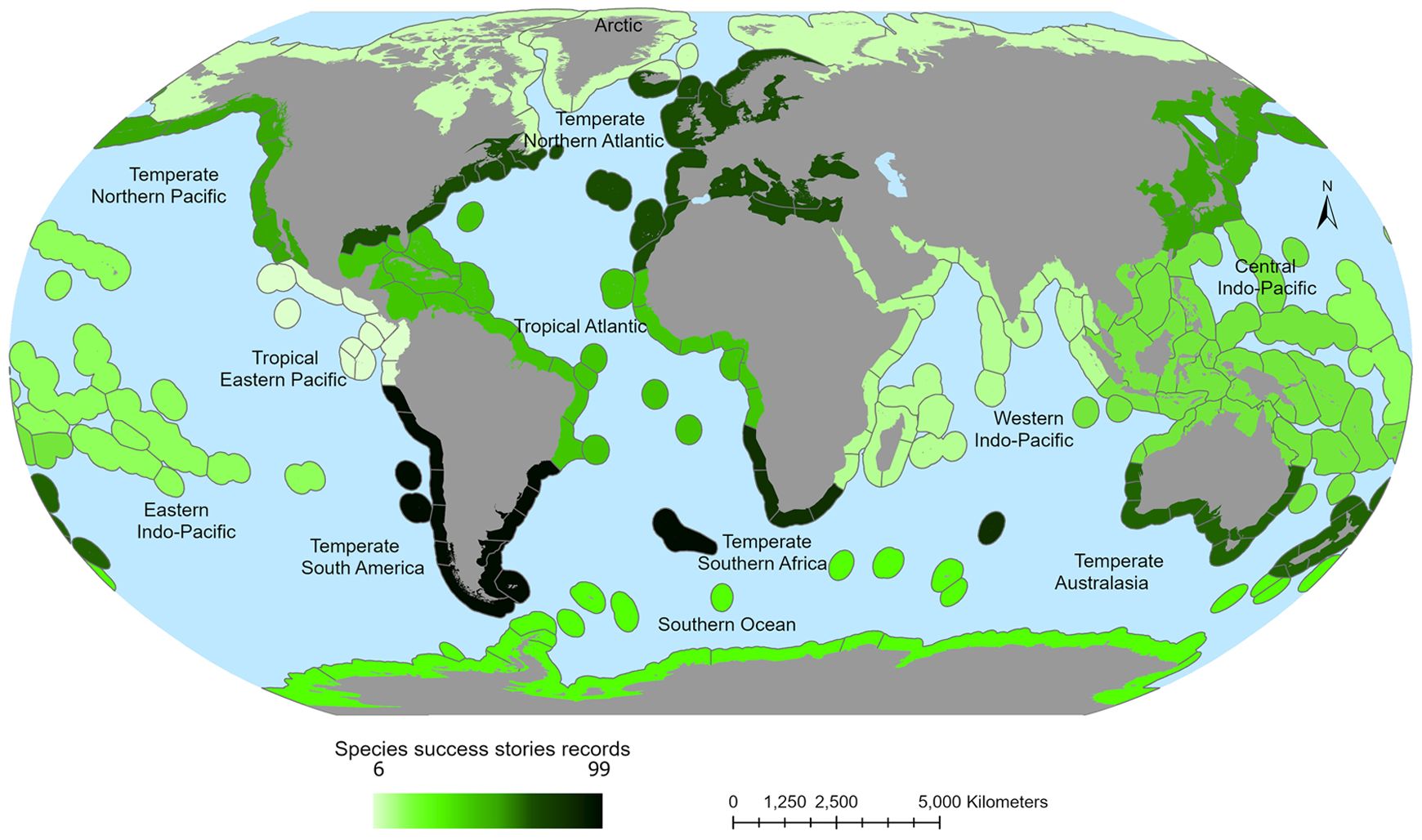
Figure 2 Spatial distribution of success stories in the twelve marine realms, sensu Spalding et al. (2007).
Management measures were predominantly local (Figure 1C); 48% of the cases were actually implemented, whereas 52% were only pilot cases. A temporal pattern in the prevalence of actual implementation of conservation measures versus pilot cases was detected, with the latter increasing drastically in the 2000s and 2010s (Supplementary Figure 7). The harbor porpoise was the most commonly targeted species, followed by the black-browed albatross (Thalassarche melanophris) and the white-chinned petrel (Procellaria aequinoctialis) (Figure 1D).
More than half of the target species in these studies were classified as ‘Least Concern’ by the IUCN, whereas only 5% were ‘Critically Endangered’ and 17% ‘Endangered’; remarkably, only three cases of ‘Data Deficient’ species were reported (Figure 3A). Most population trends for which a trend was available were classified as declining (Figure 3B).
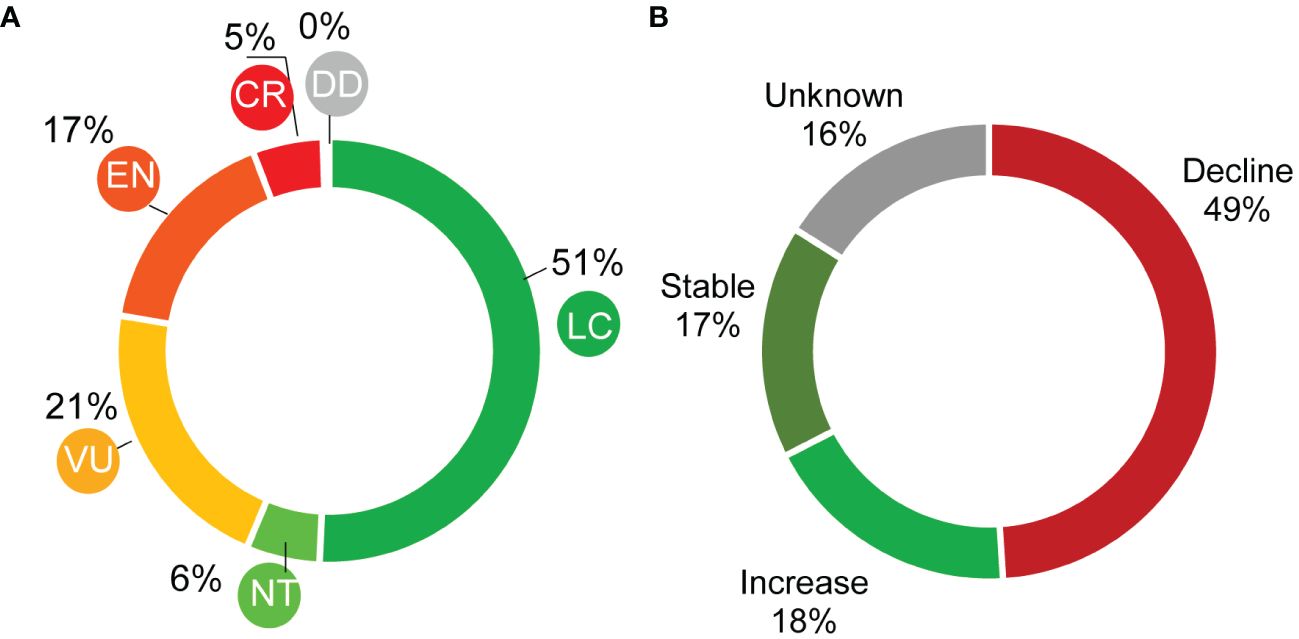
Figure 3 (A) The IUCN Red List categories of the species reported in success stories (CR: Critically Endangered, EN: Endangered, VU: Vulnerable, LC: Least Concern, DD: Data Deficient). (B) The regional population trends of the species reported in success stories according to the IUCN Red Lists assessments.
The most commonly reported management measure was bycatch reduction (57%), followed by the establishment of MPAs (15%) and invasive species management (6%) (Figure 4A). The frequency of the types of management measures in success stories significantly differed by taxonomic group (chi-square test; p < 0.0001) (Figure 5). For seabirds and marine mammals, bycatch reduction measures were by far the most commonly reported, whereas, for elasmobranchs and large Osteichthyes, the establishment of MPAs was the most common measure. In the 1980s and early 1990s, the establishment of MPAs was the main reported management measure, but in the following decades bycatch reduction measures have been dominant (Supplementary Figure 8).
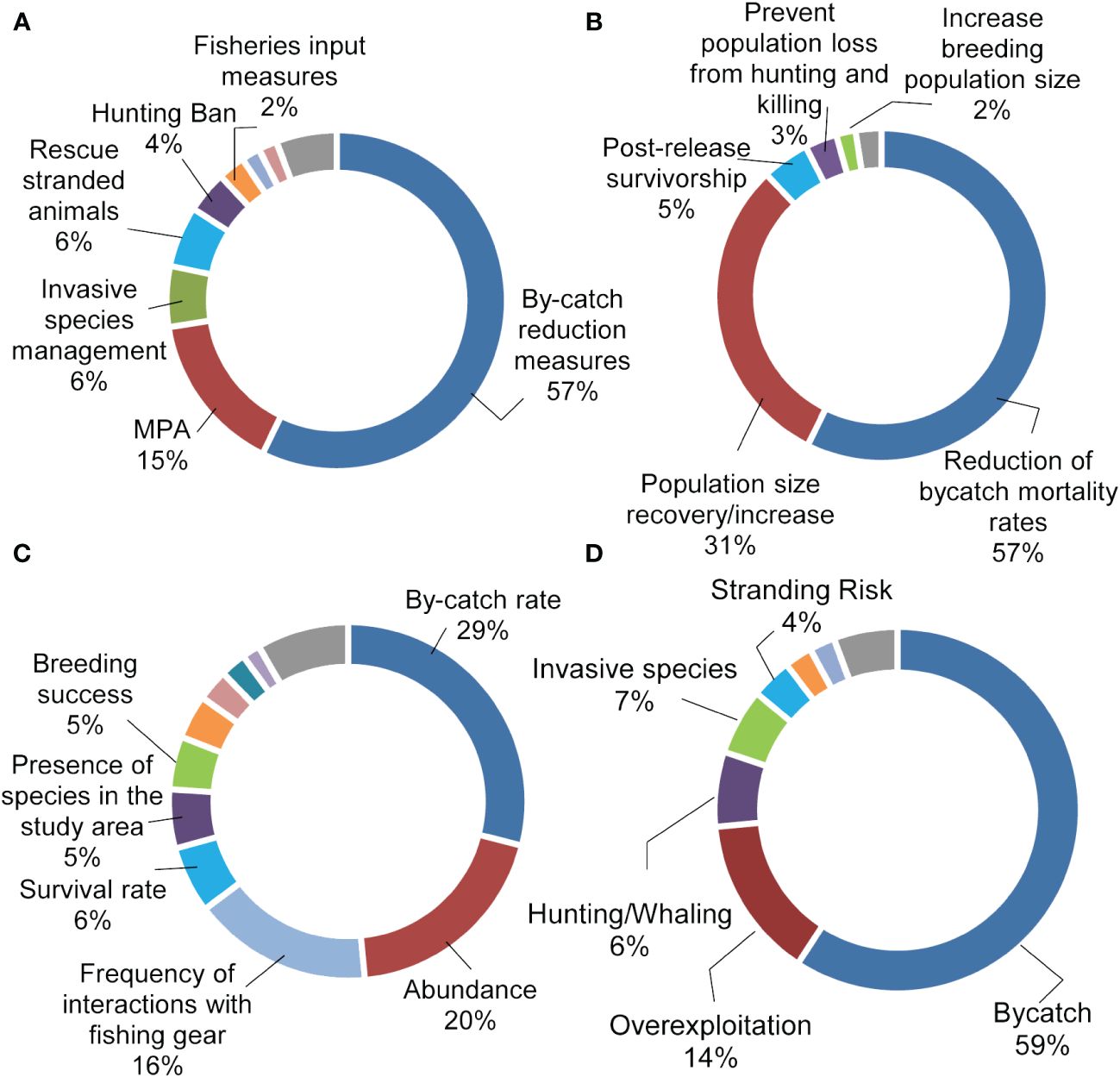
Figure 4 Summary of success stories including (A) share of the management measures, (B) conservation actions targets, (C) indicators of project success, and (D) threat mitigated.
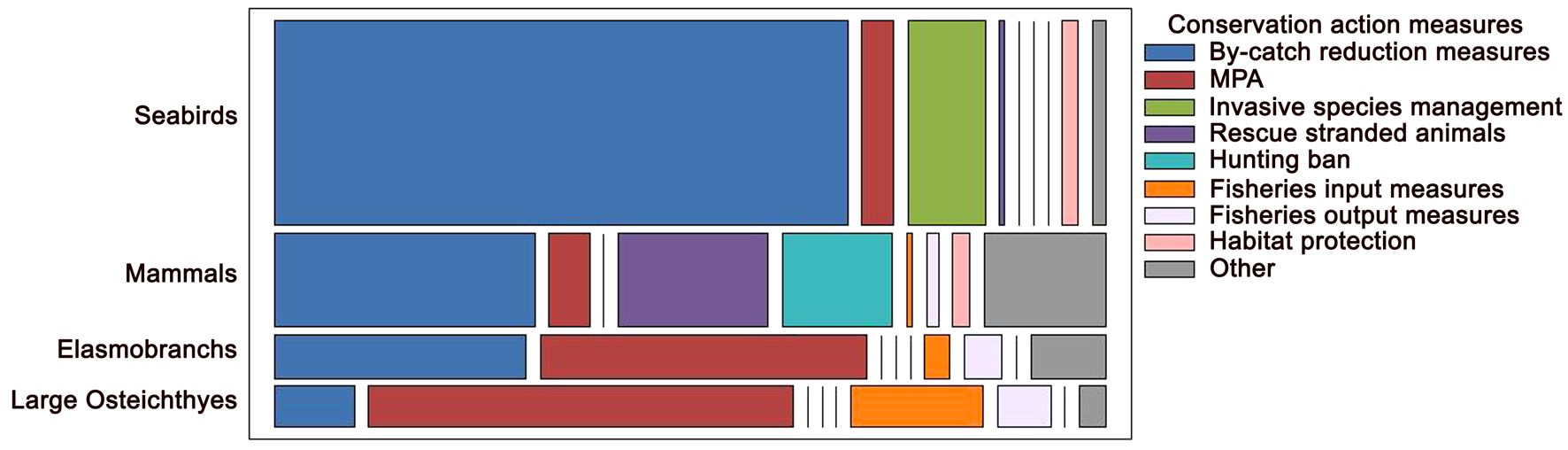
Figure 5 Type of management measures reported in success stories by taxonomic group (Key: the bar size is proportional to the number of success stories).
In terms of conservation objectives, the most common was the reduction of bycatch mortality rates (57%), followed by population size recovery/increase (31%). Other objectives, with a frequency below 5%, included: (i) increased post-release survival, (ii) reduction of intended killing or hunting, and (iii) increase of breeding population size (Figure 4B).
Various indicators were used to assess population status and the effectiveness of management, bycatch rates being the most common one (29%), followed by abundance (20%), frequency of interactions with fishing gear (other than bycatch) (16%), and survival rate (6%) (Figure 4C). The most common threat reported was bycatch (59%), followed by overexploitation (14%), hunting/whaling (6%), and invasive species (7%) (Figure 4D).
Success stories of pilot bycatch mitigation measures were four times higher than those of institutional implementation. Cox et al. (2007) acknowledged the significant progress in reducing the bycatch of marine mammals, sea turtles, and seabirds through fishing gear modifications, but underlined the challenge of transferring the efficacy of pilot mitigation measures to operational fisheries. They highlighted the collaboration among scientists, resource managers, and fishing industries, complemented by a mixture of outreach, robust enforcement, pre- and post-implementation monitoring, and economic incentives as key factors for the success of bycatch reduction measures in fisheries. Examples of implemented bycatch mitigation measures, guided by evidence-based research, include the adoption of seabird and dolphin bycatch reduction measures by all major tuna Regional Fishery Management Organizations, which are responsible for the management of over 90% of tuna fishing in the global oceans (Jiménez et al., 2020), and by the Australian Fisheries Management Authority (Koopman et al., 2018) and U.S. NOAA Fisheries.
The stakeholders involved in success stories were mostly central authorities and governmental agencies, followed by research institutions, fishing industry representatives, local NGOs, and multilateral governance instruments (Supplementary Figure 9). Miller et al. (2018) highlighted the benefits of multi-stakeholder project processes involving national and international actors. These collaborations allow for the pooling of knowledge and resources from diverse stakeholders to address complex sustainability challenges. As regularly reported in success stories, collaboration among stakeholders, local communities, and scientists are crucial factors for the success of conservation actions. For example, Lambert (2002) presented the case of the grey seal in Britain as a success story resulting from collaboration among multilateral organizations (i.e., IUCN and the European Parliament), governmental agencies, local community associations, nature conservation bodies, and NGOs. Local ecological knowledge and feedback from concerned stakeholders can provide useful insights on the status of top predator populations and options of pressures’ mitigation (e.g., Sáenz-Arroyo et al., 2005; Coll et al., 2014) and improve the chances of translating experimental measures into effective mitigation in commercial fisheries, e.g., for bycatch reduction (Cox et al., 2007). Education was highlighted in many success stories as playing a vital role in conservation efforts, ensuring that stakeholders, including local communities and industry sectors, are aware of the importance of sustainable practices (Huang, 2011).
In success stories, the effective establishment and function of MPAs were attributed to a combination of social factors, effective self-enforcement by local stakeholders, good compliance, and widespread support from local communities (Guidetti et al., 2008; Aburto-Oropeza et al., 2011; Jaiteh et al., 2016; Speed et al., 2019). Furthermore, continuous monitoring of an area allows for the adaptation of management measures over time. In the case of the Madeira Natural Park and the Desertas Islands, the success of the Mediterranean monk seal (Monachus monachus) conservation project (see also Box 2) was attributed to active patrols and environmental educational programs (Pires and Neves, 2001). Hamilton et al. (2011) highlighted the success of community-based MPAs, which achieved positive outcomes despite their small size due to well-designed plans and effective enforcement.
Securing benefits for local economies (e.g., through ecotourism) contributed to success. For example, shark diving is a rapidly growing industry that benefits not only the diving industry directly but also other economic aspects of a community (Huveneers et al., 2017). Cisneros-Montemayor et al. (2013) determined that the global shark diving industry generates $314 million per year, supporting 10,000 jobs. Also, whale sharks (Rhincodon typus) in the Philippines serve as a great example of sustainable integrated coastal governance: destructive fishing has decreased, and shark abundance has increased due to the implementation of alternative livelihood management actions based on whale shark tourism (Lowe et al., 2019). On the other hand, Mustika et al. (2020) argued that fishers in Indonesia, a biodiversity hotspot facing significant fishing pressure, do not directly benefit from shark and ray tourism. Consequently, overfishing remains a top threat to shark populations in the region.
The time required for recovery varies among species depending on their life-history characteristics and can extend beyond a century for some long-lived top predators (Lotze et al., 2011; Duarte et al., 2020). The duration of the implemented measures (excluding pilots) in the reviewed success cases was 22yr on average (median: 15yr), confirming that adequate time is needed before a significant positive result can be ascertained. Therefore, despite the substantial increase in MPAs coverage (a ten-fold increase since 2000) and the implementation of other management measures in recent years, tangible conservation outcomes for top predators are yet to be observed.
7 The way forward - recommendations
Despite all claims by global and regional policy and management organizations, the dichotomous approach to the conservation (as synonyms of management ensuring a long-term sustainable use/conservation) of the marine environment has played a key role in adoption of measures effectively halting the decline of some top predators or biodiversity in general. On the one hand the complex and not fully understood relationships between the myriad of marine ecosystem elements and on the other the systematic governmental or supra-governmental approach that is strictly sectoral, have undermined the actual application of the EBM. To move a step further towards devising efficient solutions for the conservation of top predators and biodiversity as a whole, we need to embrace more holistic and adaptive approaches to decision-making (Elliott and O’Higgins, 2020). The full understanding of socio-ecological and economical elements is difficult too given the complex interconnections between human activities and ecosystems. The Drivers-Pressures-State-Impact-Response model of intervention (DPSIR model family) is typically best suited to look at some of these links and complexities. However, elements of the ecosystem (e.g., top predators) may represent an important natural capital asset, contributing to both the structure and functioning of the marine ecosystem and delivering of societal goods and benefits (in the human domain). Hence, Elliott and O’Higgins (2020) proposed the DAPSI(W)R(M), a more holistic DPSIR-derived framework including benefits offered by nature. Further analyses of the application of this extended framework, including positive impacts on human welfare, resulted in a more comprehensive integrated model (Figure 6A), which links natural and social sciences with governance and management (Elliott, 2023), accounts for cumulative impacts across natural and social systems under a risk management framework (Stelzenmüller et al., 2018; 2020), and includes conservation as a key management measure ensuring that humans can live ‘in harmony with nature’ (see (R(M)) in Figure 6A). Figure 6B Lists all elements of the DAPSI(W)R(M) matrix that are deemed relevant to marine top predators, according to our analysis on marine mammals, marine birds, elasmobranchs and bony fish. Details on specific Responses (measures) can be found in the reviewed papers.
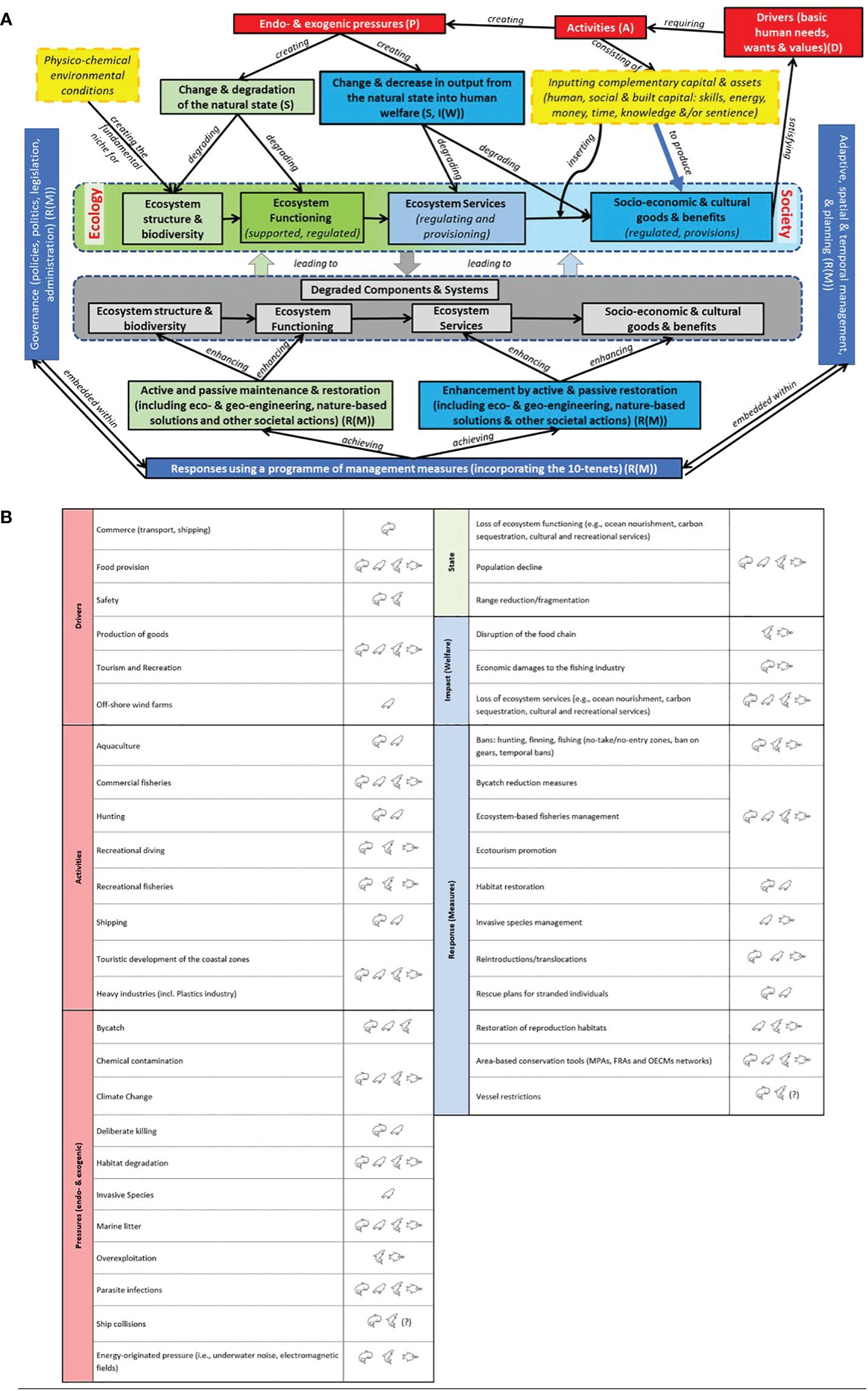
Figure 6 (A) The socio-ecological system unifying the DAPSI(W)R(M) framework, the means of degrading the natural system and recovery management measures, and the ecological structure and functioning of ecosystem services and societal goods and benefits continuum (from Elliott, 2023); (B) elements of the DAPSI(W)R(M) matrix relevant to marine top predators, according to this review (the right column shows reviewed taxa: marine mammals, marine birds, elasmobranchs, fish).
Biodiversity loss in marine ecosystems has far-reaching consequences that extend beyond the context of these top-down or bottom-up effects (McCauley et al., 2015). Defaunation can disrupt cross-system connectivity (McCauley et al., 2012a, 2012b) and undermine ecosystem stability (Britten et al., 2014). Moreover, the depletion of genetic diversity in top predator populations can reduce resilience and adaptive potential in changing environmental conditions (Heithaus et al., 2013).
The recovery of marine animal populations can be a slow and complex process (Jackson et al., 2001; Worm et al., 2006; Lotze et al., 2011). Rebuilding and restoring efforts can be successful (e.g., Bowen and Iverson, 2020) but may require sustained conservation measures and robust ecosystem-based management approaches accounting for all key ecological interactions (Pandolfi et al., 2011). Recognizing the historical role of top predators in ecosystems is a crucial initial step toward their recovery, as their past abundance and baseline may exceed estimates based on recent survey data.
In general, EBM efforts should incorporate all essential ingredients for successful implementation: accounting for ecological connections, making the best use of scientific knowledge, implementing adaptive and integrated management (and monitoring) at appropriate spatial and temporal scales, involving all relevant stakeholders, accounting for the dynamic nature of ecosystems, recognizing socio-ecological links by reflecting societal choices, and acknowledging the overall uncertainty linked to the large inherent variability of any ecosystem (Long et al., 2015).
In the following subsections we offer key recommendations to guide science-based effective conservation actions, which are summarized in Table 2.

Table 2 Summary of recommendations to guide science-based implementation of effective conservation actions.
7.1 Lessons learned from success and unsuccessful stories
The systematic review of success stories highlighted that the conservation of marine top predators requires, more than for other species or habitats, a combination of robust knowledge, education and outreach, specific and adaptive management measures, high-level stakeholder involvement (including enforcement agencies). Bycatch reduction measures and the establishment of well-managed MPAs were the most commonly reported successful actions, depending on the characteristics of the taxa. Social factors, community involvement, and securing economic benefits to local communities were critical drivers for success.
For many top predators, the primary causes of their decline are well-understood, and in many cases, effective management measures have been implemented and led to reversed trends (e.g., marine mammals that have been generally protected from hunts since mid-1980s-early 1990s; see also Boxes 1, 2). The 481 success stories identified by our review underscore the existence of a valuable knowledge base that can assist marine managers and decision-makers in taking action to reverse the decline of top predators under a holistic EBM approach aiming at the recovery of the entire marine ecosystem.
Success requires political will to implement the necessary management measures (e.g., whaling moratorium or rigorous setting of exploitation quotas). However, challenges remain when the interests at stake are much larger and linked to societal demands (e.g., fishery-related impacts on top predators and more recent intensified use of the marine system for energy, transport, and food), and the transferring of tested mitigation measures to operational fisheries addressing bycatch and overfishing remains unresolved. Our analysis shows that bycatch is by far the highest priority issue; when mitigation measures (technological, operational, and socioeconomic ones) are implemented, they become the most successful tool to reverse the decline of top predators. Compared to other direct mortalities (e.g., collisions with ships), bycatch is easier to be monitored/quantified and mitigated. Yet, implementing bycatch mitigation and monitoring policies is moving at too slow a pace, both at global and regional levels. Bycatch is an issue for which relevant authorities already have many off-the-shelf solutions. Even though the issue will likely require adaptive management because mitigation measures often work well in the short- but not the long-term, this challenge does not justify inaction. In line with the DAPSI(W)R(M) framework, a governance solution could be to equally direct funding opportunities to develop innovative methods and technologies and test other operational and effective socio-economic management tools, as these two fields are complementary.
The relatively low number of studies on the implementation of conservation measures compared to funded pilot research studies since the 2000s may indicate an increasing preference for investment on environmental research rather than on management (including large-scale implementation of technical measures) (see Figure S7). The inability to translate mitigation options identified in pilot studies into large-scale management may directly result from such unbalance or on the fact that solutions based on local tests (i.e., geographically and temporally limited) are not easily exportable or they do not work overtime. The failure to scale up may be also a consequence of the lack of codesign with appropriate stakeholders and/or limited ability to communicate benefits. In this regard, a useful open-source tool evaluating the applicability of existing mitigation and management measures and fact-checking their usefulness is the ‘Conservation Evidence’ initiative. This is a free reliable information source, built by the Department of Zoology of the University of Cambridge (UK), which is intended to inform decisions on conservation and restoration of biodiversity, by providing a comprehensive synthesis of known conservation measures for major taxonomic groups and an evaluation on their actual effectiveness.
There are different types of stakeholders, and their contribution is highly variable. In success stories, central authorities and governmental agencies were directly involved, highlighting the importance of roles and legitimacy. Conservation needs everyone’s contribution but can seldom be achieved without the involvement of competent authorities.
The overall trends from studies indicate that gaining the whole context is fundamental and that it is essential that we understand “what” and “when” we are measuring from the standpoint of population dynamics. The example of the combined use of the IUCN Green status and the IUCN Red listing categories (Box 1) helps us look at the whole context slightly differently. It provides a more realistic way to weigh and interpret the increases and declines of top predators. For example, very depleted populations may increase relatively rapidly if the environmental conditions allow and there are no biological constraints, leading to an excessively positive interpretation. In contrast, populations near or at carrying capacity may decrease or fluctuate in abundance for purely natural reasons. Abundance should always be a high-priority indicator, and a combination of genetic modeling and historical data may help roughly estimate pre-exploitation levels of top predators (e.g., Romero et al., 2022). If no information is available on the historical trajectories of a population, it is essential to consider increases in the light of implemented conservation measures (e.g., the ban of driftnets for large pelagic fish) (UNGA, 1990a, b; UNGA 1992) and the potential ecological benefit on the concerned species.
7.2 State-of-the-art research tools for the best scientific advice
Reinforcing effective monitoring of marine top predators and combining traditional and novel monitoring and modeling strategies is crucial for understanding the dynamics and ecological significance of these species within their marine ecosystems and to identify cause-effect links between human pressures and biodiversity. Long-term monitoring is imperative for these usually long-lived species. While traditional methods play a vital role in this pursuit, incorporating Local Ecological Knowledge (Sáenz-Arroyo et al., 2005; Maynou et al., 2011; Coll et al., 2014), and other novel approaches can greatly enhance our knowledge (Louzao et al., 2009; Ramos et al., 2009; Giménez et al., 2017). Technological advancements have paved the way for innovative tools, such as cheaper and smaller biologging devices (e.g., GPS tags, accelerometers, video cameras), which can be attached to animals to collect high-resolution data on their behavior and habitat use. This invaluable information encompasses diving patterns, prey preferences, and environmental interactions, enabling researchers to gain insights into predator-prey dynamics and the effects of environmental changes (Ramírez et al., 2020; Giménez et al., 2021a). Moreover, the integration of genetic techniques, such as eDNA analysis (e.g., Baker et al., 2018; Ferrari et al., 2018; Mariani et al., 2021; Suarez-Bregua et al., 2022; Valsecchi et al., 2023), or the consideration of publicly available information sourced from digital media (e.g., Sbragaglia et al., 2024), can offer useful complementary information on elusive species. By harnessing the power of both traditional and novel approaches, scientists can bolster monitoring efforts and uncover critical insights into the lives of marine top predators, ultimately aiding in the conservation and management of these species.
One of the biggest caveats when studying the effect of anthropogenic impacts on top predators is quantitative long-term data on human activities. Precise knowledge of human activities at meaningful temporal and geographical scales is often unavailable. This jeopardizes our ability to detect the impacts on top predators and inform management and conservation when needed. To reconcile the biodiversity perspective with the human-related pressures, the implementation of systematic conservation planning (Pressey and Bottrill, 2009) for marine spatial prioritization has been consistently recommended by marine scientists (Katsanevakis et al., 2020). This offers a transparent, comprehensive framework for guiding conservation efforts such as the location, configuration, and management of MPAs to achieve operational targets for ecological components while minimizing the socio-economic costs of use restrictions (Mazor et al., 2014; Yates et al., 2015; Afán et al., 2018; Giménez et al., 2021b). A comprehensive approach is required to support the conservation and resilience of top predators, as these species rely on the whole ecosystem at very large spatial and temporal scales. Despite the worldwide promotion of ecosystem-based approaches, many regions lack specific measures targeting top predators, such as the mandatory utilization of suitable bycatch reduction devices. European Union Member States must adopt programs of measures to attain a GES under the MSFD. These programs should include management actions aimed at safeguarding top predators.
Author contributions
CF: Conceptualization, Supervision, Visualization, Writing – original draft, Writing – review & editing, Project administration. TF: Conceptualization, Writing – original draft, Writing – review & editing. JB-P: Conceptualization, Writing – original draft, Writing – review & editing. MC: Conceptualization, Writing – original draft, Writing – review & editing. AF: Conceptualization, Writing – original draft, Writing – review & editing. JG: Conceptualization, Writing – original draft, Writing – review & editing. YS: Writing – original draft, Data curation, Visualization. MP: Writing – original draft, Writing – review & editing. CC: Writing – original draft. SB: Writing – original draft, Writing – review & editing. EF-C: Writing – original draft, Writing – review & editing. SF: Writing – original draft, Writing – review & editing. OG-G: Writing – original draft, Writing – review & editing. AV: Writing – original draft, Writing – review & editing. ML: Writing – original draft, Writing – review & editing. AP: Writing – original draft. SR: Conceptualization, Writing – original draft, Writing – review & editing. FR: Writing – original draft. JR: Writing – original draft. DR: Writing – review & editing. FS: Writing – original draft, Writing – review & editing. VS: Writing – original draft. SK: Conceptualization, Project administration, Visualization, Writing – original draft, Writing – review & editing.
Funding
The author(s) declare financial support was received for the research, authorship, and/or publication of this article. This research was supported by the European Union’s Horizon 2020 research and innovation programme HORIZON-CL6-2021-BIODIV-01-04 under grant agreements No 101059877 “GES4SEAS - Achieving Good Environmental Status for maintaining ecosystem SErvices, by ASsessing integrated impacts of cumulative pressures” and No 101060072 “ACTNOW” - Advancing understanding of cumulative impacts on European marine biodiversity, ecosystem functions and services for human wellbeing”. AF (and IECS.ltd participation in GES4SEAS) was supported by UK Research and Innovation (grant no. 10050522). JG was supported by the Spanish National Program Juan de la Cierva-Formación (FJC2019-040016-I). OG-G was supported by a Margarita Salas grant funded by the Spanish Ministry of Universities and the European Union Next Generation EU/PRTR, as well as the recovery, transformation, and resilience plan, and the complementary call for applications for grants for the requalification of the Spanish university system for the period 2021-2023.
Acknowledgments
This work acknowledges the accreditation of the ‘Severo Ochoa Center of Excellence’ to ICM-CSIC (CEX2019-000928-S).
Conflict of interest
Author AF was employed by The International Estuarine & Coastal specialists Ltd.
The remaining Authors declare that the research was conducted in the absence of any commercial or financial relationships that could be construed as a potential conflict of interest.
The author(s) declared that they were an editorial board member of Frontiers, at the time of submission. This had no impact on the peer review process and the final decision.
Publisher’s note
All claims expressed in this article are solely those of the authors and do not necessarily represent those of their affiliated organizations, or those of the publisher, the editors and the reviewers. Any product that may be evaluated in this article, or claim that may be made by its manufacturer, is not guaranteed or endorsed by the publisher.
Supplementary material
The Supplementary Material for this article can be found online at: https://www.frontiersin.org/articles/10.3389/fmars.2024.1282091/full#supplementary-material
References
Aarts G., Brasseur S., Poos J. J., Schop J., Kirkwood R., Kooten T. V., et al. (2019). Top-down pressure on a coastal ecosystem by harbor seals. Ecosphere 10 (1), e02538. doi: 10.1002/ecs2.2538
Aarts G., MacKenzie M., McConnell B., Fedak M., Matthiopoulos J. (2008). Estimating space-use and habitat preference from wildlife telemetry data. Ecography 31, 140–160. doi: 10.1111/j.2007.0906-7590.05236.x
Aburto-Oropeza O., Erisman B., Galland G. R., Mascareñas-Osorio I., Sala E., Ezcurra E. (2011). Large recovery of fish biomass in a no-take marine reserve. PloS One 6, e23601. doi: 10.1371/journal.pone.0023601
ACCOBAMS. (2021). Estimates of abundance and distribution of cetaceans, marine mega-fauna and marine litter in the Mediterranean Sea from 2018-2019 surveys. ACCOBAMS - ACCOBAMS Survey Initiative Project (Monaco), 177.
Aceves-Bueno E., Adeleye A., Feraud M., Huang Y., Tao M., Yang Y., et al. (2017). The accuracy of citizen science data: A quantitative review. Bull. Ecol. Soc. America 98, 278–290. doi: 10.1002/bes2.1336
Afán I., Giménez J., Forero M. G., Ramírez F. (2018). An adaptive method for identifying marine areas of high conservation priority. Conserv. Biol. 32, 1436–1447. doi: 10.1111/cobi.13154
Aguilar A., Lowry L. (2010) Monachus monachus. The IUCN Red List of Threatened Species 2010 (IUCN SSC Pinniped Specialist Group Mediterranean regional assessment) (Accessed 27 March 2023).
Akçakaya H. R., Bennett E. L., Brooks T. M., Grace M. K., Heath A., Hedges S., et al. (2018). Quantifying species recovery and conservation success to develop an IUCN Green List of Species. Conserv. Biol. 32, 1128–1138. doi: 10.1111/cobi.13112
Albonetti L., Maiello G., Cariani A., Carpentieri P., Ferrari A., Sbrana A., et al. (2023). DNA metabarcoding of trawling bycatch reveals diversity and distribution patterns of sharks and rays in the central Tyrrhenian Sea. ICES J. Mar. Sci. 80, 664–674. doi: 10.1093/icesjms/fsad022
Amundin M., Carlström J., Thomas L., Carlén I., Koblitz J., Teilmann J., et al. (2022). Estimating the abundance of the critically endangered Baltic Proper harbour porpoise (Phocoena phocoena) population using passive acoustic monitoring. Ecol. Evol. 12, e8554. doi: 10.1002/ece3.8554
Anastasopoulou A., Fortibuoni T. (2019). “Impact of Plastic Pollution on Marine Life in the Mediterranean Sea,” in Handbook of Environmental Chemistry, vol. 5 . Eds. Stock F., Reifferscheid G., Brennholt N., Kostianaia E. (Springer Nature, Switzerland), 1–12. doi: 10.1007/698_2019_421
Andrews A. J., Di Natale A., Bernal-Casasola D., Aniceti V., Onar V., Queslati T., et al. (2022). Exploitation history of Atlantic bluefin tuna in the eastern Atlantic and Mediterranean - insights from ancient bones. ICES J. Mar. Sci. 79, 247–262. doi: 10.1093/icesjms/fsab261
Andrzejaczek S., Lucas T. C., Goodman M. C., Hussey N. E., Armstrong A. J., Carlisle A., et al. (2022). Diving into the vertical dimension of elasmobranch movement ecology. Sci. Adv. 8, eabo1754. doi: 10.1126/sciadv.abo1754
Angiolillo M., Fortibuoni T. (2020). Impacts of marine litter on Mediterranean reef systems: from shallow to deep waters. Front. Mar. Sci. 7. doi: 10.3389/fmars.2020.581966
Arcos J., Oro D. (2002). Significance of nocturnal purse seine fisheries for seabirds: a case study off the Ebro Delta (NW Mediterranean). Mar. Biol. 141, 277–286. doi: 10.1007/s00227-002-0828-3
Astarloa A., Glennie R., Chust G., García-Baron I., Boyra G., Martínez U., et al. (2021). Niche segregation mechanisms in marine apex predators inhabiting dynamic environments. Divers. Distrib. 27, 799–815. doi: 10.1111/ddi.13229
Astarloa A., Louzao M., Boyra G., Martinez U., Rubio A., Irigoien X., et al. (2019). Identifying main interactions in marine predator–prey networks of the Bay of Biscay. ICES J. Mar. Sci. 76, 2247–2259. doi: 10.1093/icesjms/fsz140
Atwood T. B., Connolly R. M., Ritchie E. G., Lovelock C. E., Heithaus M. R., Hays G. C., et al. (2015). Predators help protect carbon stocks in blue carbon ecosystems. Nat. Clim. Change 5, 1038–1045. doi: 10.1038/nclimate2763
Atwood T. B., Hammill E. (2018). The importance of marine predators in the provisioning of ecosystem services by coastal plant communities. Front. Plant Sci. 9. doi: 10.3389/fpls.2018.01289
Authier M., Dorémus G., Van Canneyt O., Boubert J. J., Gautier G., Doray M., et al. (2018). Exploring change in the relative abundance of marine megafauna in the Bay of Biscay 2004-2016. Prog. Oceanogr. 166, 159–167. doi: 10.1016/j.pocean.2017.09.014
Bailey H., Brookes K. L., Thompson P. M. (2014). Assessing environmental impacts of offshore wind farms: lessons learned and recommendations for the future. Aquat. Biosyst. 10, 1–13. doi: 10.1186/2046-9063-10-8
Baker C. S., Steel D., Nieukirk S., Klinck H. (2018). Environmental DNA (eDNA) from the wake of the whales: droplet digital PCR for detection and species identification. Front. Mar. Sci. 5. doi: 10.3389/fmars.2018.00133
Bakker J., Wangensteen O. S., Chapman D. D., Boussarie G., Buddo D., Guttridge T. L., et al. (2017). Environmental DNA reveals tropical shark diversity in contrasting levels of anthropogenic impact. Sci. Rep. 7, 1–11. doi: 10.1038/s41598-017-17150-2
Banks J., Lea M. A., Wall S., McMahon C. R., Hindell M. A. (2014). Combining bio-logging and fatty acid signature analysis indicates spatio-temporal variation in the diet of the southern elephant seal, Mirounga leonina. J. Exp. Mar. Biol. Ecol. 450, 79–90. doi: 10.1016/j.jembe.2013.10.024
Barbraud C., Weimerskirch H. (2003). Climate and density shape population dynamics of a marine top predator. Pro. R. Soc Lond. B. 270, 2111–2116. doi: 10.1098/rspb.2003.2488
Barcelona Convention (2008). Decision IG.17/6 on Implementation of the ecosystem approach to the management of human activities that may affect the Mediterranean marine and coastal environment. Athens, Greece: UNEP MAP.
Barcelona Convention (2012). Decision IG.20/4. on Implementing MAP ecosystem approach roadmap: Mediterranean Ecological and Operational Objectives, Indicators and Timetable for implementing the ecosystem approach roadmap adopted by the Contracting parties to the Barcelona Convention. Athens, Greece: UNEP MAP.
Barcelona Convention (2013). Decision IG. 21/3 on The Ecosystem Approach including adopting definitions of Good Environmental Status (GES) and targets. Athens, Greece: UNEP MAP.
Barcelona Convention (2016). Decision IG.22/7 on Integrated Monitoring and Assessment Programme of the Mediterranean Sea and Coast and Related Assessment Criteria. Athens, Greece: UNEP MAP.
Baum J. K., Worm B. (2009). Cascading top-down effects of changing oceanic predator abundances. J. Anim. Ecol. 78, 699–714. doi: 10.1111/j.1365-2656.2009.01531.x
Berg T., Fürhaupter K., Teixeira H., Uusitalo L., Zampoukas N. (2015). The marine strategy framework directive and the ecosystem-based approach - pitfalls and solutions. Mar. pollut. Bull. 96, 18–28. doi: 10.1016/j.marpolbul.2015.04.050
Bertrand A., Josse E. (2000). Acoustic estimation of longline tuna abundance. ICES J. Mar. Sci. 57, 919–926. doi: 10.1006/jmsc.2000.0579
Bevilacqua S., Airoldi L., Ballesteros E., Benedetti-Cecchi L., Boero F., Bulleri F., et al. (2021). Mediterranean rocky reefs in the Anthropocene: present status and future concerns. Adv. Mar. Biol. 89, 1–51. doi: 10.1016/bs.amb.2021.08.001
BirdLife International (2021) Puffinus mauretanicus (Europe assessment). The IUCN Red List of Threatened Species 2021 (Accessed 20 July 2023).
Bjørndal T. (2021). The Northeast Atlantic and Mediterranean Bluefin Tuna Fishery: Back from the Brink? (Centre for Applied Research at NHH).
Bode A., Saavedra C., Álvarez-González M., Arregui M., Arbelo M., Fernández A., et al. (2022). Trophic position of dolphins tracks recent changes in the pelagic ecosystem of the Macaronesian region (NE Atlantic). Mar. Ecol. Prog. Ser. 699, 167–180. doi: 10.3354/meps14176
Bom R. A., Brader A., Batsleer J., Poos J. J., van der Veer H. W., Van Leeuwen A. (2022). A long-term view on recent changes in abundance of common skate complex in the North Sea. Mar. Biol. 169, 146. doi: 10.1007/s00227-022-04132-w
Bond A. L., Jones I. L. (2009). A practical introduction to stable-isotope analysis for seabird biologists: approaches, cautions and caveats. Mar. Ornithol. 37, 183–188.
Booth C. G., Guilpin M., Darias-O’Hara A. K., Ransijn J. M., Ryder M., Rosen D., et al. (2023). Estimating energetic intake for marine mammal bioenergetic models. Conserv. Physiol. 11, coac083. doi: 10.1093/conphys/coac083
Borchers D. L., Laake J. L., Southwell C., Paxton C. G. M. (2006). Accommodating unmodeled heterogeneity in double-observer distance sampling surveys. Biometrics 62, 372–378. doi: 10.1111/j.1541-0420.2005.00493.x
Borrell A., Gazo M., Aguilar A., Raga J. A., Degollada E., Gozalbes P., et al. (2021). Niche partitioning amongst northwestern Mediterranean cetaceans using stable isotopes. Prog. Oceanog. 193, 102559. doi: 10.1016/j.pocean.2021.102559
Borthwick A. G. (2016). Marine renewable energy seascape. Engineering 2, 69–78. doi: 10.1016/J.ENG.2016.01.011
Brasseur S. M. J. M., Reijnders P. J. H., Cremer J., Meesters E., Kirkwood R., Jensen L. F., et al. (2018). Echoes from the past: Regional variations in recovery within a harbour seal population. PloS One 13, e0189674. doi: 10.1371/journal.pone.0189674
Brasseur S. M. J. M., van Polanen Petel T. D., Gerrodette T., Meesters E. H. W. G., Reijnders P. J. H., Aarts G. (2015). Rapid recovery of Dutch grey seal colonies fueled by immigration. Mar. Mam. Sci. 31, 405–426. doi: 10.1111/mms.12160
Braun C. D., Lezama-Ochoa N., FarChadi N., Arostegui M., Alexander M., Allyn A., et al. (2023). Widespread habitat loss and redistribution of marine top predators in a changing ocean. Sci. Adv. 9, e2718. doi: 10.1126/sciadv.adi2718
Britten G. L., Dowd M., Minto C., Ferretti F., Boero F., Lotze H. K. (2014). Predator decline leads to decreased stability in a coastal fish community. Ecol. Lett. 17, 1518–1525. doi: 10.1111/ele.12354
Brooks K., Rowat D., Pierce S. J., Jouannet D., Vely M. (2010). Seeing spots: photo-identification as a regional tool for whale shark identification. Western Indian Ocean J. Mar. Sci. 9, 185–194.
Buckland S. T., Anderson D. R., Burnham K. P., Laake J. L., Borchers D. L., Thomas L. (2004). Advanced distance sampling: estimating abundance of biological populations (OUP Oxford). doi: 10.1093/oso/9780198507833.001.0001
Budd A. M., Cooper M. K., Le Port A., Schils T., Mills M. S., Deinhart M. E., et al. (2021). First detection of critically endangered scalloped hammerhead sharks (Sphyrna lewini) in Guam, Micronesia, in five decades using environmental DNA. Ecol. Indic. 127, 107649. doi: 10.1016/j.ecolind.2021.107649
Burgess M. G., Becker S. L. (2022). Good and bad news for ocean predators. Science 378, 596–597. doi: 10.1126/science.add0342
Campbell L. M., Fairbanks L., Murray G., Stoll J. S., D’Anna L., Bingham J. (2021). From Blue Economy to Blue Communities: reorienting aquaculture expansion for community wellbeing. Mar. Policy 124, 104361. doi: 10.1016/j.marpol.2020.104361
Carter M. I. D., Boehme L., Cronin M. A., Duck C. D., Grecian W. J., Hastie G. D., et al. (2022). Sympatric seals, satellite tracking and protected areas: habitat-based distribution estimates for conservation and management. Front. Mar. Sci. 9. doi: 10.3389/fmars.2022.875869
Cavanagh R. D., Gibson C. (2007). Overview of the Conservation Status of Cartilaginous Fishes (Chondrichthyans) in the Mediterranean Sea (Gland, Switzerland and Malaga, Spain: IUCN), vi + 42. doi: 10.2305/IUCN.CH.2007.MRA.3.en
CBD (Convention on Biological Diversity) (2021). First draft of the post-2020 global biodiversity framework. Open ended Working Group on the post-2020 global biodiversity framework, Third meeting Online, 23 August – 3 September 2021. Document CBD/WG2020/3/3. 12.
Charrassin J. B., Hindell M., Rintoul S. R., Roquet F., Sokolov S., Biuw M., et al. (2008). Southern Ocean frontal structure and sea-ice formation rates revealed by elephant seals. Proc. Natl. Acad. Sci. U.S.A. 105, 11634–11639. doi: 10.1073/pnas.0800790105
Chatzimentor A., Doxa A., Katsanevakis S., Mazaris A. (2023). Are Mediterranean marine threatened species at high risk by climate change? Glob. Change Biol. 29, 1809–1821. doi: 10.1111/gcb.16577
Chavez-Rosales S., Palka D. L., Garrison L. P., Josephson E. A. (2019). Environmental predictors of habitat suitability and occurrence of cetaceans in the western North Atlantic Ocean. Sci. Rep. 9, 5833. doi: 10.1038/s41598-019-42288-6
Christensen V., Coll M., Steenbeek J., Buszowski J., Chagaris D., Walters. C. J. (2014). Representing variable habitat quality in a spatial food web model. Ecosystems 17, 1397–1412. doi: 10.1007/s10021-014-9803-3
Christensen V., Ferdaña Z., Steenbeek J. (2009). Spatial optimization of protected area placement incorporating ecological, social and economical criteria. Ecol. Modell. 220, 2583–2593. doi: 10.1016/j.ecolmodel.2009.06.029
Christensen V., Walters C. (2004). Ecopath with Ecosim: methods, capabilities and limitations. Ecol. Modell. 72, 109–139. doi: 10.1016/j.ecolmodel.2003.09.003
Claudet J., Fraschetti S. (2010). Human-driven impacts on marine habitats: a regional meta-analysis in the Mediterranean Sea. Biol. Conserv. 143, 2195–2206. doi: 10.1016/j.biocon.2010.06.004
Coll M., Albo-Puigserver M., Navarro J., Palomera I., Dambacher J. M. (2019b). Who is to blame? Plausible pressures on small pelagic fish population changes in the NW Mediterranean Sea. Mar. Ecol. Prog. Ser. 617-618, 277–294. doi: 10.3354/meps12591
Coll M., Carreras M., Ciércoles C., Cornax M. J., Gorelli G., Morote E., et al. (2014). Assessing fishing and marine biodiversity changes using fishers’ perceptions: the Spanish Mediterranean and Gulf of Cadiz case study. PloS One 9, e85670. doi: 10.1371/journal.pone.0085670
Coll M., Navarro J., Palomera I. (2013). Ecological role, fishing impact, and management options for the recovery of a Mediterranean endemic skate by means of food web models. Biol. Conserv. 157, 108–120. doi: 10.1016/j.biocon.2012.06.029
Coll M., Pennino M. G., Sole J., Steenbeek J., Bellido J. M. (2019a). Predicting marine species distributions: complementarity of food-web and Bayesian hierarchical modelling approaches. Ecol. Modell. 405, 86–101. doi: 10.1016/j.ecolmodel.2019.05.005
Coll M., Piroddi C., Steenbeek J., Kaschner K., Aguzzi J., Ben Rais Lasram F., et al. (2010). The biodiversity of the Mediterranean sea: estimates, patterns, and threats. PloS One 5, e11842. doi: 10.1371/journal.pone.0011842
Coll M., Steenbeek J., Lasram F. B. R., Mouillot D., Cury P. (2015). “Low hanging fruits” for conservation of marine vertebrate species at risk in the Mediterranean Sea. Glob. Ecol. Biogeogr. 24, 226–239. doi: 10.1111/geb.12250
Coll M., Steenbeek J., Pennino M. G., Buszowski J., Kaschner K., Lotze H. K., et al. (2020). Advancing global ecological modelling capabilities to simulate future trajectories of change in marine ecosystems. Front. Mar. Sci. 7. doi: 10.3389/fmars.2020.567877
Cooch E., White G. C. (2008) Program MARK, “A gentle introduction”. Available online at: http://www.phidot.org/software/mark/docs/book/.
Course G. P., Pierre J., Howell B. K. (2020). What’s in the Net? Using camera technology to monitor, and support mitigation of, wildlife bycatch in fisheries (WWF).
Cox T. M., Lewison R. L., Žydelis R., Crowder L. B., Safina C., Read A. J. (2007). Comparing effectiveness of experimental and implemented bycatch reduction measures: The ideal and the real. Conserv. Biol. 21, 1155–1164. doi: 10.1111/j.1523-1739.2007.00772.x
Cox S., Martell S., Walters C., Essington T., Kitchell J., Boggs C., et al. (2002). Reconstructing ecosystem dynamics in the central Pacific Ocean 1952–1998. II. doi: 10.1139/f02-138
Currey-Randall L. M., Cappo M., Simpfendorfer C. A., Farabaugh N. F., Heupel M. R. (2020). Optimal soak times for baited remote underwater video station surveys of reef-associated elasmobranchs. PloS One 15, e0231688. doi: 10.1371/journal.pone.0231688
Curtin R., Prellezo R. (2010). Understanding marine ecosystem based management: a literature review. Mar. Policy 34, 821–830. doi: 10.1016/j.marpol.2010.01.003
Curtis T. H., McCandless C. T., Carlson J. K., Skomal G. B., Kohler N. E., Natanson L. J., et al. (2014). Seasonal distribution and historic trends in abundance of white sharks, carcharodon carcharias, in the Western North Atlantic ocean. PloS One 9, e99240. doi: 10.1371/journal.pone.0099240
Cury P. M., Boyd I. L., Bonhommeau S., Anker-Nilssen T., Crawford R. J. M., Furness R. W., et al. (2011). Global seabird response to forage fish depletion–one-third for the birds. Science 334, 1703–1706. doi: 10.1126/science.1212928
Danovaro R. (2003). Pollution threats in the Mediterranean Sea: an overview. Chem. Ecol. 19, 15–32. doi: 10.1080/0275754031000081467
de Mutsert K., Coll M., Steenbeek J., Ainsworth C. H., Buszowski J., Chagaris D., et al. (2023). “Advances in spatial-temporal coastal and marine ecosystem modelling using Ecopath with Ecosim and Ecospace,” in 2nd Edition of the Treatise on Coastal Science. Reference Module in Earth Systems and Environmental Sciences, vol. 2023 . Ed. U. B. Scharler D. (Elsevier), ISBN: ISBN 9780124095489.
Descamps S., Ramírez F. (2021). Species and spatial variation in the effects of sea ice on Arctic seabird populations. Divers. Distrib. 27, 2204–2217. doi: 10.1111/ddi.13389
de Sousa L. L., Silva S. M., Xavier R. (2019). DNA metabarcoding in diet studies: Unveiling ecological aspects in aquatic and terrestrial ecosystems. Environ. DNA 1, 199–214. doi: 10.1002/edn3.27
de Vooys C. G. N., Brasseur S. M. J. M., van der Meer J., Reijnders P. J. H. (2012). Analyses of four centuries of bounty hunting on seals in Zeeland, SW-Netherlands. Lutra 55, 55–65.
den Heyer C. E., Bowen W. D., Dale J., Gosselin J. F., Hammill M. O., Johnston D. W., et al. (2021). Contrasting trends in gray seal (Halichoerus grypus) pup production throughout the increasing Northwest Atlantic metapopulation. Mar. Mam. Sci. 37, 611–630.
Dias M. P., Martin R., Pearmain E. J., Burfield I. J., Small C., Phillips R. A., et al. (2019). Threats to seabirds: a global assessment. Biol. Conserv. 237, 525–537. doi: 10.1016/j.biocon.2019.06.033
Doughty C. E., Roman J., Faurby S., Wolf A., Haque A., Bakker E. S., et al. (2016). Global nutrient transport in a world of giants. P. Nat. Acad. Sci. 113, 868–873. doi: 10.1073/pnas.1502549112
Duarte C. M., Agusti S., Barbier E., Britten G. L., Castilla J. C., Gattuso J.-P., et al. (2020). Rebuilding marine life. Nature 580, 39–51. doi: 10.1038/s41586-020-2146-7
Duffy-Anderson J. T., Stabeno P., Andrews A. G., Cieciel K., Deary A., Farley E., et al. (2019). Responses of the northern Bering Sea and southeastern Bering Sea pelagic ecosystems following record-breaking low winter sea-ice. Geophysical Res. Let. 46 (16), 9833–9842. doi: 10.1029/2019GL083396
Dulvy N. K., Allen D. J., Ralph G. M., Walls R. H. L. (2016). The Conservation Status of Sharks, Rays and Chimaeras in the Mediterranean Sea [Brochure] (Malaga, Spain: IUCN). Available at: https://portals.iucn.org/library/sites/library/files/documents/Rep-2016-024.pdf.
Dulvy N. K., Fowler S. L., Musick J. A., Cavanagh R. D., Kyne P. M., Harrison L. R., et al. (2014). Extinction risk and conservation of the world’s sharks and rays. eLife 3, e00590. doi: 10.7554/eLife.00590
Dulvy N. K., Simpfendorfer C. A., Davidson L. N., Fordham S. V., Bräutigam A., Sant G., et al. (2017). Challenges and priorities in shark and ray conservation. Curr. Biol. 27, R565–R572. doi: 10.1016/j.cub.2017.04.038
Dunn D. C., Boustany A. M., Halpin P. N. (2011). Spatio-temporal management of fisheries to reduce by-catch and increase fishing selectivity. Fish Fisheries 12, 110–119. doi: 10.1111/j.1467-2979.2010.00388.x
EC (European Commission) (2018). Marine Strategy Framework Directive (MSFD) Common Implementation Strategy. Reporting on the 2018 update of articles 8, 9 & 10 for the Marine Strategy Framework Directive (Brussels: MSFD Guidance Document 14, October 2019 version) Luxembourg: Publications Office of the European Union, 75.
EC (European Commission) (2023). Communication from the Commission to the European Parliament, the Council, the European Economic and Social Committee and the Committee of the Regions - EU Action Plan: Protecting and restoring marine ecosystems for sustainable and resilient fisheries (Brussels, Luxembourg: Publications Office of the European Union), 24.
Elliott M. (2023). Editorial - Marine Ecosystem Services and Integrated Management: “There’s a crack, a crack in everything, that’s how the light gets in”! Mar. Poll. Bull. 193, 115117. doi: 10.1016/j.marpolbul.2023.115177
Elliott M., O’Higgins T. G. (2020). “From DPSIR the DAPSI(W)R(M) Emerges … a Butterfly – ‘protecting the natural stuff and delivering the human stuff’,” in Ecosystem-based management, ecosystem services and aquatic biodiversity: Theory, tools and applications. Eds. O’Higgins T., Lago M., DeWitt T. H. (Springer, Amsterdam), 61–86. doi: 10.1007/978-3-030-45843-0_4
Erauskin-Extramiana M., Chust G., Arrizabalaga H., Cheung W. W., Santiago J., Merino G., et al. (2023). Implications for the global tuna fishing industry of climate change-driven alterations in productivity and body sizes. Global Planetary Change 222, 104055. doi: 10.1016/j.gloplacha.2023.104055
Estes J. A., Doak D. F., Springer A. M., Williams T. M. (2009). Causes and consequences of marine mammal population declines in southwest Alaska: a food-web perspective. Phil. Trans. R. Soc. B 364, 1647–1658. doi: 10.1098/rstb.2008.0231
Estes J. A., Heithaus M., McCauley D. J., Rasher D. B., Worm B. (2016). Megafaunal impacts on structure and function of ocean ecosystems. Annu. Rev. Environ. Resour. 41, 83–116. doi: 10.1146/annurev-environ-110615-085622
European Commission. (2017). Commission Decision (EU) 2017/848 of 17 May 2017 laying down criteria and methodological standards on good environmental status of marine waters and specifications and standardised methods for monitoring and assessment, and repealing Decision 2010/477/EU. Off. J. Eur. Union L 125 (Luxembourg), 43–74. http://data.europa.eu/eli/dec/2017/848/oj.
European Council. (1992). Council Regulation (EEC) No 345/92 of 27 January 1992 amending for the eleventh time Regulation (EEC) No 3094/86 laying down certain technical measures for the conservation of fishery resources. Off. J. L 042 (Luxembourg), 15–23. http://data.europa.eu/eli/reg/1992/345/oj.
European Council. (1997). Council Regulation (EC) No 894/97 of 29 April 1997 laying down certain technical measures for the conservation of fishery resources. Off. J. L 132 (Luxembourg), 1–27. https://eur-lex.europa.eu/eli/reg/1997/894/oj.
European Union. (2019). Regulation (EU) 2019/1241 of the European Parliament and of the Council of 20 June 2019 on the conservation of fisheries resources and the protection of marine ecosystems through technical measures, amending Council Regulations (EC) No 1967/2006, (EC) No 1224/2009 and Regulations (EU) No 1380/2013, (EU) 2016/1139, (EU) 2018/973, (EU) 2019/472 and (EU) 2019/1022 of the European Parliament and of the Council, and repealing Council Regulations (EC) No 894/97, (EC) No 850/98, (EC) No 2549/2000, (EC) No 254/2002, (EC) No 812/2004 and (EC) No 2187/2005. Off. J. Eur. Union L (Luxembourg), 105–201. http://data.europa.eu/eli/reg/2019/1241/oj.
Faure N., Manel S., Macé B., Arnal V., Guellati N., Holon F., et al. (2023). An environmental DNA assay for the detection of Critically Endangered angel sharks (Squatina spp.). Aquat. Conservation: Mar. Freshw. Ecosyst. 33 (10), 1088–97. doi: 10.1002/aqc.3954
Ferrari A., Tinti F., Bertucci Maresca V., Velonà A., Cannas R., Thasitis I., et al. (2018). Natural history and molecular evolution of demersal Mediterranean sharks and skates inferred by comparative phylogeographic and demographic analyses. PeerJ 6, e5560. doi: 10.7717/peerj.5560
Ferretti F., Myers R. A., Serena F., Lotze H. K. (2008). Loss of large predatory sharks from the Mediterranean sea. Conserv. Biol. 22, 952–964. doi: 10.1111/j.1523-1739.2008.00938.x
Ferretti F., Worm B., Britten G. L., Heithaus M. R., Lotze H. K. (2010). Patterns and ecosystem consequences of shark declines in the ocean. Ecol. Lett. 13, 1055–1071. doi: 10.1111/j.1461-0248.2010.01489.x
Field I. C., Tillett B. J., Charters R., Johnson G. J., Buckworth R. C., Meekan M. G., et al. (2013). Distribution, relative abundance and risks from fisheries to threatened Glyphis sharks and sawfishes in northern Australia. Endanger. Species Res. 21, 171–180. doi: 10.3354/esr00513
Follesa M. C., Marongiu M. F., Zupa W., Bellodi A., Cau A., Cannas R., et al. (2019). Spatial variability of Chondrichthyes in the northern Mediterranean. Sci. Mar. 83, 81–100. doi: 10.3989/scimar.2019.83S1
Foote A. D., Thomsen P. F., Sveegaard S., Wahlberg M., Kielgast J., Kyhn L. A., et al. (2012). Investigating the potential use of environmental DNA (eDNA) for genetic monitoring of marine mammals. PloS One 7, e41781. doi: 10.1371/journal.pone.0041781
Fortuna C. M., Cañadas A., Holcer D., Brecciaroli B., Donovan G. P., Lazar B., et al. (2018). The coherence of the European union marine natura 2000 network for wide-ranging charismatic species: A mediterranean case study. Front. Mar. Sci. 5. doi: 10.3389/fmars.2018.00356
Fortuna C. M., Kell L., Holcer D., Canese S., Filidei E., Mackelworth P., et al. (2014). Summer distribution and abundance of the giant devil ray (Mobula mobular) in the Adriatic Sea: baseline data for an iterative management framework. Scientia Marina 78, 277–237. doi: 10.3989/scimar.2014.78n2
Fortune S. M. E., Trites A. W., Mayo C. A., Rosen D. A., Hamilton P. K. (2013). Energetic requirements of North Atlantic right whales and the implications for species recovery. Mar. Ecol. Prog. Ser. 478, 253–272. doi: 10.3354/meps10000
Fossi M. C., Vlachogianni T., Galgani F., Degli Innocenti F., Zampetti G., Leone G. (2020). Assessing and mitigating the harmful effects of plastic pollution: the collective multi-stakeholder driven Euro-Mediterranean response. Ocean Coast. Manage. 184, 105005. doi: 10.1016/j.ocecoaman.2019.105005
Franco A., Elliott M., Franzoi P., Nunn A., Hänfling B., Colclough S., et al. (2022). “Appendix A. Study Methods I: Field Equipment, Sampling and Methods,” in Fish and Fisheries in Estuaries: A Global Perspective. Eds. Whitfield A. K., Able K. W., Blaber S. J. M., Elliott M. (John Wiley & Sons, Oxford, UK), 874–940, ISBN: ISBN 978-1444336672.
Franco A., Okane C., Elliott M., Haines R., Sharkey W. (2021) Coordinated assessments of marine species and habitats under the Birds and Habitats Directives and the Marine Strategy Framework Directive. Process and Technical review: Main Report (Final) and Annexes. A report to the European Commission, Directorate General for Environment, written by ICF in association with MRAG, University of Hull, and partners, May 2021. Available online at: https://ec.europa.eu/environment/marine/publications/pdf/BHD-MFSD_coordination%20study_Main_Final.pdf.
Frederiksen M., Anker-Nilssen T., Beaugrand G., Wanless S. (2013). Climate, copepods and seabirds in the boreal Northeast Atlantic - current state and future outlook. Glob. Change Biol. 19, 364–372. doi: 10.1111/gcb.12072
Fretwell P. T., Staniland I. J., Forcada J. (2014). Whales from Space: Counting Southern Right Whales by Satellite. PloS One 9 (2), e88655. doi: 10.1371/journal.pone.0088655
Fulton E. A. (2010). Approaches to end-to-end ecosystem models. J. Mar. Syst. 81, 171–183. doi: 10.1016/j.jmarsys.2009.12.012
Fulton E. A. (2011). Interesting times: winners, losers, and system shifts under climate change around Australia. ICES J. Mar. Sci. 68, 1329–1342. doi: 10.1093/icesjms/fsr032
Fulton E. A., Bax N. J., Bustamante R. H., Dambacher J. M., Dichmont C., Dunstan P. K., et al. (2015). Modelling marine protected areas: insights and hurdles. Phil. Trans. R. Soc B 370, 20140278. doi: 10.1098/rstb.2014.0278
García-Barón I., Authier M., Caballero A., Vázquez J. A., Santos M. B., Murcia J. L., et al. (2019). Modelling the spatial abundance of a migratory predator: a call for transboundary marine protected areas. Diversity Distributions 25, 346–360. doi: 10.1111/ddi.12877
García-Barón I., Giakoumi S., Santos M. B., Granado I., Louzao M. (2021). The value of time-series data for conservation planning. J. Appl. Ecol. 58, 608–619. doi: 10.1111/1365-2664.13790
García-Barón I., Santos M. B., Saavedra C., Astarloa A., Valeiras J., Barcelona S. G., et al. (2020). Essential ocean variables and high value biodiversity areas: Targets for the conservation of marine megafauna. Ecol. Indic. 117, 106504. doi: 10.1016/j.ecolind.2020.106504
Garcia-Garin O., Borrell A., Vighi M., Aguilar A., Valdivia M., González E. M., et al. (2021). Long-term assessment of trace elements in franciscana dolphins from the Río de la Plata estuary and adjacent Atlantic waters. Sci. Total Environ. 788, 147797. doi: 10.1016/j.scitotenv.2021.147797
Garcia-Garin O., Sahyoun W., Net S., Vighi M., Aguilar A., Ouddane B., et al. (2022). Intrapopulation and temporal differences of phthalate concentrations in North Atlantic fin whales (Balaenoptera physalus). Chemosphere 300, 134453. doi: 10.1016/j.chemosphere.2022.134453
García-Vernet R., Borrell A., Víkingsson G., Halldórsson S. D., Aguilar A. (2021). Ecological niche partitioning between baleen whales inhabiting Icelandic waters. Prog. Oceanogr. 199, 102690. doi: 10.1016/j.pocean.2021.102690
Gaspar C., Giménez J., Andonegi E., Astarloa A., Chouvelon T., Franco J., et al. (2022). Trophic ecology of northern gannets Morus bassanus highlights the extent of isotopic niche overlap with other apex predators within the Bay of Biscay. Mar. Biol. 169, 105. doi: 10.1007/s00227-022-04079-y
Geelhoed S. C. V., Authier M., Pigeault R., Gilles A. (2022). “Abundance and Distribution of Cetaceans,” in OSPAR 2023: The 2023 Quality Status Report for the Northeast Atlantic (OSPAR Commission, London). Available at: https://oap.ospar.org/en/ospar-assessments/quality-status-reports/qsr-2023/indicator-assessments/abundance-distribution-cetaceans.
Genovart M., Arcos J. M., Álvarez D., McMinn M., Meier R., B. Wynn R., et al. (2016). Demography of the critically endangered Balearic shearwater: the impact of fisheries and time to extinction. J. Appl. Ecol. 53, 1158–1168. doi: 10.1111/1365-2664.12622
Giacomo A. B., Barreto J., Teixeira J. B., Oliveira L., Cajaíba L., Joyeux J. C., et al. (2021). Using drones and ROV to assess the vulnerability of marine megafauna to the Fundão tailings dam collapse. Sci. Total Environ. 800, 149302. doi: 10.1016/j.scitotenv.2021.149302
Giménez J., Arneill G. E., Bennison A., Pirotta E., Gerritsen H. D., Bodey T. W., et al. (2021a). Sexual mismatch between vessel-associated foraging and discard consumption in a marine top predator. Front. Mar. Sci. 8. doi: 10.3389/fmars.2021.636468
Giménez J., Cañadas A., de Stephanis R., Ramírez F. (2021b). Expanding protected areas to encompass the conservation of the endangered common dolphin (Delphinus delphis) in the Alboran Sea. Mar. Environ. Res. 168, 105305. doi: 10.1016/j.marenvres.2021.105305
Giménez J., Cañadas A., Ramírez F., Afán I., García-Tiscar S., Fernández-Maldonado C., et al. (2018). Living apart together: Niche partitioning among Alboran Sea cetaceans. Ecol. Indic. 95, 32–40. doi: 10.1016/j.ecolind.2018.07.020
Giménez J., Cardador L., Mazor T., Kark S., Bellido J. M., Coll M., et al. (2020). Marine protected areas for demersal elasmobranchs in highly exploited Mediterranean ecosystems. Mar. Environ. Res. 160, 105033. doi: 10.1016/j.marenvres.2020.105033
Giménez J., Gómez-Campos E., Borrell A., Cardona L., Aguilar A. (2013). Isotopic evidence of limited exchange between Mediterranean and eastern North Atlantic fin whales. Rapid Commun. Mass Spectrom. 27, 1801–1806. doi: 10.1002/rcm.6633
Giménez J., Marçalo A., Ramírez F., Verborgh P., Gauffier P., Esteban R., et al. (2017). Diet of bottlenose dolphins (Tursiops truncatus) from the Gulf of Cadiz: Insights from stomach content and stable isotope analyses. PloS One 12, e0184673. doi: 10.1371/journal.pone.0184673
Giménez J., Ramírez F., Coll M., Navarro J. (2022). “Charismatic, threatened and unknown: marine predators in the Anthropocene,” in The ocean we want: inclusive and transformative ocean science. Eds. Pelegrí J. L., Gili J. M., Martínez de Albéniz M. V., 65–67. doi: 10.20350/digitalCSIC/14066
Glad A., Monnet J. M., Pagel J., Reineking B. (2019). Importance and effectiveness of correction methods for spatial sampling bias in species with sex-specific habitat preference. Ecol. Evol. 9, 13188–13201. doi: 10.1002/ece3.5765
Gomei M., Steenbeek J., Coll M., Claudet J. (2021). 30 by 30: Scenarios to recover biodiversity and rebuild fish stocks in the Mediterranean Vol. 29 (Rome, Italy: WWF Mediterranean Marine Initiative). Available at: https://www.wwf.eu/?2248641/Scenarios-to-recover-biodiversity-and-rebuild-fish-stocks-in-the-Mediterranean-Sea.
González-Andrés C., Sánchez-Lizaso J. L., Cortés J., Pennino M. G. (2021). Predictive habitat suitability models to aid the conservation of elasmobranchs in Isla Del Coco national park (Costa Rica). J. Mar. Syst. 224, 103643. doi: 10.1016/j.jmarsys.2021.103643
Gormley A. M., Slooten E., Dawson S., Barker R. J., Rayment W., du Fresne S., et al. (2012). First evidence that marine protected areas can work for marine mammals. J. Appl. Ecol. 49, 474–480. doi: 10.1111/j.1365-2664.2012.02121.x
Grace M. K., Akçakaya H. R., Bennett E. L., Boyle M. J. W., Hilton-Taylor C., Hoffmann M., et al. (2022). The impact of spatial delineation on the assessment of species recovery outcomes. Diversity 14, 742. doi: 10.3390/d14090742
Guénette S., Heymans S. J. J., Christensen V., Trites A. W. (2006). Ecosystem models show combined effects of fishing, predation, competition, and ocean productivity on Steller sea lions (Eumetopias jubatus) in Alaska. Can. J. Fish. Aquat. Sci. 63, 2495–2517. doi: 10.1139/f06-136
Guidetti P. (2006). Marine reserves reestablish lost predatory interactions and cause community changes in rocky reefs. Ecol. Appl. 16, 963–976. doi: 10.1890/1051-0761(2006)016[0963:MRRLPI]2.0.CO;2
Guidetti P., Milazzo M., Bussotti S., Molinari A., Murenu M., Pais A., et al. (2008). Italian marine reserve effectiveness: Does enforcement matter? Biol. Conserv. 141, 699–709. doi: 10.1016/j.biocon.2007.12.013
Guisan A., Zimmermann N. E. (2000). Predictive habitat distribution models in ecology. Ecol. Modell. 135, 147–186. doi: 10.1016/S0304-3800(00)00354-9
Gutiérrez-Muñoz P., Walters A. E. M., Dolman S. J., Pierce G. J. (2021). Patterns and trends in cetacean occurrence revealed by shorewatch, a land-based citizen science program in Scotland (United Kingdom). Front. Mar. Sci. 8. doi: 10.3389/fmars.2021.642386
Haas A. R., Fedler T., Brooks E. J. (2017). The contemporary economic value of elasmobranchs in The Bahamas: Reaping the rewards of 25 years of stewardship and conservation. Biol. Conserv. 207, 55–63. doi: 10.1016/j.biocon.2017.01.007
Hagihara R., Cleguer C., Preston S., Sobtzick S., Hamann M., Shimada T., et al. (2016). Improving the estimates of abundance of dugongs and large immature and adult-sized green turtles in Western and Central Torres Strait. Report to the National Environmental Science Programme (Reef and Rainforest Research Centre Limited), 53.
Hamilton R. J., Potuku T., Montambault J. R. (2011). Community-based conservation results in the recovery of reef fish spawning aggregations in the Coral Triangle. Biol. Conserv. 144, 1850–1858. doi: 10.1016/j.biocon.2011.03.024
Hammerschlag N., Schmitz O. J., Flecker A. S., Lafferty K. D., Sih A., Atwood T. B., et al. (2019). Ecosystem function and services of aquatic predators in the Anthropocene. Trends Ecol. Evol. 34, 369–383. doi: 10.1016/j.tree.2019.01.005
Hammond P. S. (1986). Estimating the size of naturally marked whale populations using capture-recapture techniques. IWC, Cambridge, UK: Reports of the International Whaling Commission, Vol. 8. 253–282.
Hammond P. S. (2010). “Estimating the abundance of marine mammals,” in Marine Mammal Ecology and Conservation: a Handbook of Techniques. Eds. Boyd I. L., Bowen W. D., Iverson S. (Oxford University Press, New York, NY), 42–67.
Hammond P. S., Lacey C., Gilles A., Viquerat S., Boerjesson P., Herr H., et al. (2021). Estimates of cetacean abundance in European Atlantic waters in summer 2016 from the SCANS-III aerial and shipboard surveys. SCANS-III report.
Hammond P. S., Macleod K., Berggren P., Borchers D. L., Burt M. L., Cañadas A., et al. (2013). Cetacean abundance and distribution in European Atlantic shelf waters to inform conservation and management. Biol. Conserv. 164, 107–122. doi: 10.1016/j.biocon.2013.04.010
Hazen E. L., Abrahms B., Brodie S., Carroll G., Jacox M. G., Savoca M. S., et al. (2019). Marine top predators as climate and ecosystem sentinels. Front. Ecol. Environ. 17, 565–574. doi: 10.1002/fee.2125
Heithaus M. R., Frid A., Wirsing A. J., Dill L. M., Fourqurean J. W., Burkholder D., et al. (2013). State-dependent risk-taking by green sea turtles mediates top-down effects of tiger shark intimidation in a marine ecosystem. J. Anim. Ecol. 82, 78–89. doi: 10.1111/j.1365-2656.2007.01260.x
Heithaus M. R., Frid A., Wirsing A. J., Worm B. (2008). Predicting ecological consequences of marine top predator declines. Trends Ecol. Evol. 23, 202–210. doi: 10.1016/j.tree.2008.01.003
Hernández-Camacho C. J., Bakker V. J., Aurioles-Gamboa D., Laake J., Gerber L. R. (2015). The use of surrogate data in demographic population viability analysis: a case study of California sea lions. PloS One 10, e0139158. doi: 10.1371/journal.pone.0139158
Heupel M. R., Simpfendorfer C. A. (2002). Estimation of mortality of juvenile blacktip sharks, Carcharhinus limbatus, within a nursery area using telemetry data. Can. J. Fish. Aquat. Sci. 59, 624–632. doi: 10.1139/f02-036
Hirzel A. H., Le Lay G. (2008). Habitat suitability modelling and niche theory. J. Appl. Ecol. 45, 1372–1381. doi: 10.1111/j.1365-2664.2008.01524.x
Holland K., Meyer C., Potemra J., Holland M. (2022). Ocean depth–temperature profiles for operational oceanography from a shark-borne transmitter. Anim. Biotelemetry 10, 34. doi: 10.1186/s40317-022-00306-x
Huang H. W. (2011). Bycatch of high sea longline fisheries and measures taken by Taiwan: Actions and challenges. Mar. Policy 35, 712–720. doi: 10.1016/j.marpol.2011.02.012
Hughes B. J., Martin G. R., Giles A. D., Reynolds S. J. (2017). Long-term population trends of Sooty Terns Onychoprion fuscatus: Implications for conservation status. Popul. Ecol. 59, 213–224. doi: 10.1007/s10144-017-0588-z
Hui F. K. (2016). BORAL—Bayesian ordination and regression analysis of multivariate abundance data in R. Methods Ecol. Evol. 7, 744–750. doi: 10.1111/2041-210X.12514
Hutchinson G. E. (1957). Concluding remarks. Cold Spring Harbor Symp. Quantitative Biol. 22, 415–427. doi: 10.1101/SQB.1957.022.01.039
ICCAT. (2017). Report of the 2017 ICCAT bluefin stock assessment meeting (Madrid, Spain: ICCAT Secretariat). Available at: https://www.iccat.int/Documents/SCRS/DetRep/BFT_SA_ENG.pdf.
ICCAT. (2020). Report of the 2020 second ICCAT intersessional meeting of the bluefin tuna species group (Madrid, Spain: ICCAT Secretariat). Available at: https://www.iccat.int/Documents/Meetings/Docs/2020/REPORTS/2020_2_BFT_ENG.pdf.
ICES (2017). Manual of the IBTS North Eastern Atlantic Surveys, Vol. 15. 92. doi: 10.17895/ices.pub.3519
ICES (2021). Working Group on Bycatch of Protected Species (WGBYC) Vol. 3 (ICES Scientific Reports), 168. doi: 10.17895/ices.pub.9256
ICES. (2020). Manual for the North Sea International Bottom Trawl Surveys, Vol. 102. doi: 10.17895/ices.pub.7562
ICES. (2022). Working Group on Marine Mammal Ecology (WGMME) (ICES Sci. Rep. Report). doi: 10.17895/ices.pub.20448942.v1
IJsseldijk L. L., Camphuysen C. J., Keijl G. O., Troost G., Aarts G. (2021). Predicting Harbor porpoise strandings based on near-shore sightings indicates elevated temporal mortality rates. Front. Mar. Sci 8. doi: 10.3389/fmars.2021.668038
IUCN. (2023) The IUCN Red List of Threatened Species. Version 2022-2. Available online at: https://www.iucnredlist.org (Accessed January-February 2023).
IWC. (1999). Report of the Scientific Committee, Annex N. The revised management procedure (RMP) for baleen whales. J. Cetacean Res. Manage. 1, 251–258.
Jackson J. B., Kirby M. X., Berger W. H., Bjorndal K. A., Botsford L. W., Bourque B. J., et al. (2001). Historical overfishing and the recent collapse of coastal ecosystems. Science 293, 629–637. doi: 10.1126/science.1059199
Jaiteh V. F., Lindfield S. J., Mangubhai S., Warren C., Fitzpatrick B., Loneragan N. R. (2016). Higher abundance of marine predators and changes in fishers’ behaviour following spatial protection within the world’s biggest shark fishery. Front. Mar. Sci. 3. doi: 10.3389/fmars.2016.00043
Jaramillo-Legorreta A., Cardenas-Hinojosa G., Nieto-Garcia E., Rojas-Bracho L., Ver Hoef J., Moore J., et al. (2017). Passive acoustic monitoring of the decline of Mexico’s critically endangered vaquita. Conserv. Biol. 31, 183–191. doi: 10.1111/cobi.12789
Jarić I., Roll U., Arlinghaus R., Belmaker J., Chen Y., China V., et al. (2020). Expanding conservation culturomics and iecology from terrestrial to aquatic realms. PloS Biol. 18, e3000935. doi: 10.1371/journal.pbio.3000935
Jeanniard-du-Dot T., Trites A. W., Arnould J. P., Speakman J. R., Guinet C. (2017). Activity-specific metabolic rates for diving, transiting, and resting at sea can be estimated from time-activity budgets in free-ranging marine mammals. Ecol. Evol. 7, 2969–2976. doi: 10.1002/ece3.2546
Jenrette J. F., Jenrette J. L., Truelove N. K., Moro S., Dunn N. I., Chapple T. K. (2023). Detecting Mediterranean white sharks with environmental DNA. Oceanography 36, 87–89. doi: 10.5670/oceanog.2023.s1.28
Jepson P. D., Deaville R., Barber J. L., Aguilar À., Borrell A., Murphy S., et al. (2016). PCB pollution continues to impact populations of orcas and other dolphins in European waters. Sci. Rep. 6, 18573. doi: 10.1038/srep18573
Jiménez S., Domingo A., Winker H., Parker D., Gianuca D., Neves T., et al. (2020). Towards mitigation of seabird bycatch: Large-scale effectiveness of night setting and Tori lines across multiple pelagic longline fleets. Biol. Conserv. 247, 108642. doi: 10.1016/j.biocon.2020.108642
JRC (Joint Research Centre) (2018). Lists of species and habitats for 2018 MSFD reporting. Marine Strategy Framework Directive (MSFD) Common Implementation Strategy, 19th Meeting of the Working Group on Good Environmental Status (WG GES), 22 March 2018 (Brussels, Luxembourg: Publications Office of the European Union), 7.
Juan-Jordá M. J., Murua H., Arrizabalaga H., Merino G., Pacoureau N., Dulvy N. K. (2022). Seventy years of tunas, billfishes, and sharks as sentinels of global ocean health. Science 378, eabj0211. doi: 10.1126/science.abj0211
Juhel J. B., Marques V., Fernández A. P., Borrero-Pérez G. H., Martinezguerra M. M., Valentini A., et al. (2021). Detection of the elusive Dwarf sperm whale (Kogia sima) using environmental DNA at Malpelo island (Eastern Pacific, Colombia). Ecol. Evol. 11, 2956. doi: 10.1002/ece3.7057
Karamanlidis A. A., Adamantopoulou S., Tounta E., Dendrinos P. (2019) Monachus monachus (Eastern Mediterranean subpopulation). The IUCN Red List of Threatened Species 2019 (Accessed 04 January 2024).
Karamanlidis A. A., Dendrinos D., Fernandez de Larrinoa P., Gücü A. C., Johnson W. M., Kiraç C. O., et al. (2015). Status, biology, threats and conservation priorities of the Mediterranean monk seal Monachus monachus. Mammal Rev. doi: 10.1111/mam.12053
Karamanlidis A. A., Dendrinos P., Fernandez de Larrinoa P., Kıraç C. O., Nicolaou H., Pires R. (2023) Monachus monachus. The IUCN Red List of Threatened Species 2023 (Accessed 04 January 2024).
Katsanevakis S., Coll M., Fraschetti S., Giakoumi S., Goldsborough D., Mačić V., et al. (2020). Twelve recommendations for advancing marine conservation in European and contiguous seas. Front. Mar. Sci. 7. doi: 10.3389/fmars.2020.565968
Katsanevakis S., Stelzenmüller V., South A., Sørensen T. K., Jones P. J., Kerr S., et al. (2011). Ecosystem-based marine spatial management: review of concepts, policies, tools, and critical issues. Ocean Coast. Manage. 54, 807–820. doi: 10.1016/j.ocecoaman.2011.09.002
Kelly B. C., Ikonomou M. G., Blair J. D., Surridge B., Hoover D., Grace R., et al. (2009). Perfluoroalkyl contaminants in an arctic marine food web: Trophic magnification and wildlife exposure. Environ. Sci. Technol. 43, 4037–4043. doi: 10.1021/es9003894
Koopman M., Boag S., Tuck G. N., Hudson R., Knuckey I., Alderman R. (2018). Industry-based development of effective new seabird mitigation devices in the southern Australian trawl fisheries. Endanger. Species Res. 36, 197–211. doi: 10.3354/esr00896
Kytinou E., Sini M., Issaris Y., Katsanevakis S. (2020). Global systematic review of methodological approaches to analyze coastal shelf food webs. Front. Mar. Sci. 7. doi: 10.3389/fmars.2020.00636
Labrousse S., Iles D., Viollat L., Fretwell P., Trathan P. N., Zitterbart D. P., et al. (2022). Quantifying the causes and consequences of variation in satellite-derived population indices: a case study of emperor penguins. Remote Sens. Ecol. Conserv. 8, 151–165. doi: 10.1002/rse2.233
Laffoley D., Dudley N., Jonas H., MacKinnon D., MacKinnon K., Hockings M., et al. (2017). An introduction to ‘other effective area-based conservation measures’ under Aichi Target 11 of the Convention on Biological Diversity: Origin, interpretation and emerging ocean issues. Aquat. Conserv: Mar. Freshw. Ecosyst. 27, 130–137. doi: 10.1002/aqc.2783
Lambert R. A. (2002). The grey seal in Britain: A twentieth century history of a nature conservation success. Environ. Hist. 8, 449–474. doi: 10.3197/096734002129342738
Lan K.-W., Wu Y.-L., Chen L.-C., Naimullah M., Lin T.-H. (2021). Effects of climate change in marine ecosystems based on the spatiotemporal age structure of top predators: A case study of bigeye tuna in the Pacific ocean. Front. Mar. Sci. 8. doi: 10.3389/fmars.2021.614594
Lauria V., Gristina M., Attrill M., Fiorentino F., Garofalo G. (2015). Predictive habitat suitability models to aid conservation of elasmobranch diversity in the central Mediterranean Sea. Sci. Rep. 5, 13245. doi: 10.1038/srep13245
Lennox R., Sbragaglia V., Vollset K., Sortland L., McClenachan L., Jarić I., et al. (2022). Digital fisheries data in the internet age: Emerging tools for research and monitoring using online data in recreational fisheries. Fish Fish. 23, 926–940. doi: 10.1111/faf.12663
Lewis A. E., Hammill M. O., Power M., Doidge D. W., Lesage V. (2009). Movement and aggregation of eastern Hudson Bay beluga whales (Delphinapterus leucas): a comparison of patterns found through satellite telemetry and Nunavik traditional ecological knowledge. Arctic 62, 13–24. doi: 10.14430/arctic109
Lloret-Lloret E., Navarro J., Giménez J., López N., Albo-Puigserver M., Pennino M. G., et al. (2020). The seasonal distribution of a highly commercial fish is related to ontogenetic changes in its feeding strategy. Front. Mar. Sci. 7. doi: 10.3389/fmars.2020.566686
Long R. D., Charles A., Stephenson R. L. (2015). Key principles of marine ecosystem-based management. Mar. Policy 57, 53–60. doi: 10.1016/j.marpol.2015.01.013
Long R. D., Charles A., Stephenson R. L. (2017). Key principles of ecosystem-based management: the fishermen’s perspective. Fish Fish. 18, 244–253. doi: 10.1111/faf.12175
Lotze H. K., Coll M., Magera M. A., Ward-Paige C., Airoldi L. (2011). Recovery of marine animal populations and ecosystems. Trends Ecol. Evol. 26, 595–605. doi: 10.1016/j.tree.2011.07.008
Lotze H. K., Lenihan H. S., Bourque B. J., Bradbury R. H., Cooke R. G., Kay M. C., et al. (2006). Depletion, degradation, and recovery potential of estuaries and coastal seas. Science 312, 1806–1809. doi: 10.1126/science.1128035
Lotze H. K., Tittensor D. P., Bryndum-Buchholz A., Eddy T. D., Galbraith E. D., et al. (2019). Global ensemble projections reveal trophic amplification of ocean biomass declines with climate change. Proc. Natl. Acad. Sci. U.S.A. 116, 12907–12912. doi: 10.1073/pnas.1900194116
Lotze H. K., Worm B. (2009). Historical baselines for large marine animals. Trends Ecol. Evol. 24, 254–262. doi: 10.1016/j.tree.2008.12.004
Louzao M., Arcos J. M., Guijarro B., Valls M., Oro D. (2011a). Seabird-trawling interactions: factors affecting species-specific to regional community utilisation of fisheries waste. Fisheries Oceanogr. 20, 263–277. doi: 10.1111/j.1365-2419.2011.00579.x
Louzao M., Aumont O., Hothorn T., Wiegand T., Weimerskirch H. (2013). Foraging in a changing environment: habitat shifts of an oceanic predator over the last half century. Ecography 36, 57–67. doi: 10.1111/j.1600-0587.2012.07587.x
Louzao M., Bécares J., Rodríguez B., Hyrenbach K. D., Ruiz A., Arcos J. M. (2009). Combining vessel-based surveys and tracking data to identify key marine areas for seabirds. Mar. Ecol. Prog. Ser. 391, 183–197. doi: 10.3354/meps08124
Louzao M., Delord K., Garcia D., Boue A., Weimerskirch H. (2012). Protecting persistent dynamic oceanographic features: transboundary conservation efforts are needed for the critically endangered Balearic shearwater. PloS One 7, e35728. doi: 10.1371/journal.pone.0035728
Louzao M., Hyrenbach K. D., Arcos J. M., Abelló P., de Sola L. G., Oro D. (2006). Oceanographic habitat of an endangered Mediterranean procellariiform: implications for marine protected areas. Ecol. Appl. 16, 1683–1695. doi: 10.1890/1051-0761(2006)016[1683:OHOAEM]2.0.CO;2
Louzao M., Navarro J., Forero M. G., Igual J. M., Genovart M., Hobson K. A., et al. (2011b). Exploiting the closest productive area: geographical segregation of foraging grounds in a critically endangered seabird. Mar. Ecol. Prog. Ser. 429, 291–301. doi: 10.3354/meps09126
Louzao M., Pinaud D., Peron C., Delord K., Wiegand T., Weimerskirch H. (2011c). Conserving pelagic habitats: seascape modelling of an oceanic top predator. J. Appl. Ecol. 48, 121–132. doi: 10.1111/j.1365-2664.2010.01910.x
Louzao M., Ruiz J., Oyarzabal I., Basterretxea M., Pedrajas A., Mugerza A., et al. (2020). Including ecosystem descriptors in current fishery data collection programmes to advance towards a holistic monitoring: Seabird abundance attending demersal trawlers. Mar. Environ. Res. 160, 105043. doi: 10.1016/j.marenvres.2020.105043
Louzao M., Valeiras J., García-Barcelona S., González-Quirós R., Nogueira E., Iglesias M., et al. (2019). Marine megafauna niche coexistence and hotspot areas in a temperate ecosystem. Continental Shelf Res. 186, 77–87. doi: 10.1016/j.csr.2019.07.013
Louzao M., Wiegand T., Bartumeus F., Weimerskirch H. (2014). Coupling instantaneous energy-budget models and behavioural mode analysis to estimate optimal foraging strategy: an example with wandering albatrosses. Movement Ecol. 2, 1–15. doi: 10.1186/2051-3933-2-8
Lunn N. J., Servanty S., Regehr E. V., Converse S. J., Richardson E., Stirling I. (2016). Demography of an apex predator at the edge of its range: impacts of changing sea ice on polar bears in Hudson Bay. Ecol. Appl. 26, 1302–1320. doi: 10.1890/15-1256
MacKenzie B. R., Mosegaard H., Rosenberg A. A. (2009). Impending collapse of bluefin tuna in the northeast Atlantic and Mediterranean. Conserv. Lett. 2, 26–35. doi: 10.1111/j.1755-263X.2008.00039.x
MacKenzie B. R., Romeo T., Addis P., Battaglia P., Consoli P., Andaloro F., et al. (2021). New historical data for long-term swordfish ecological studies in the Mediterranean Sea. Earth Syst. Sci. Data 13, 5867–5877. doi: 10.5194/essd-13-5867-2021
Mancusi C., Baino R., Fortuna C., De Sola L., Morey G., Bradai M. N., et al. (2020). MEDLEM database, a data collection on large Elasmobranchs in the Mediterranean and Black seas. Mediterr. Mar. Sci. 21, 276–288. doi: 10.12681/mms.21148
Mariani S., Fernandez C., Baillie C., Magalon H., Jaquemet S. (2021). Shark and ray diversity, abundance and temporal variation around an Indian Ocean Island, inferred by eDNA metabarcoding. Conserv. Sci. Pract. 3, e407. doi: 10.1111/csp2.407
Maynou F., Sbrana M., Sartor P., Maravelias C., Kavadas S., Damalas D., et al. (2011). Estimating trends of population decline in long-lived marine species in the Mediterranean sea based on fishers’ Perceptions. PloS One 6, e21818. doi: 10.1371/journal.pone.0021818
Mazor T., Possingham H. P., Edelist D., Brokovich E., Kark S. (2014). The crowded sea: incorporating multiple marine activities in conservation plans can significantly alter spatial priorities. PloS One 9, e104489. doi: 10.1371/journal.pone.0104489
McCauley D. J., DeSalles P. A., Young H. S., Dunbar R. B., Dirzo R., Mills M. M., et al. (2012a). From wing to wing: The persistence of long ecological interaction chains in less-disturbed ecosystems. Sci. Rep. 2, 409. doi: 10.1038/srep00409
McCauley D. J., Hazen E. L., Lewison R. L., Dunn D. C., Bailey H., Bograd S. J., et al. (2015). Marine defaunation: animal loss in the global ocean. Science 347, 1255641. doi: 10.1126/science.1255641
McCauley D. J., Micheli F., Young H. S., Tittensor D. P., Brumbaugh D. R., Madin E. M., et al. (2010). Acute effects of removing large fish from a near-pristine coral reef. Mar. Biol. 157, 2739–2750. doi: 10.1007/s00227-010-1533-2
McCauley D. J., Young H. S., Dunbar R. B., Estes J. A., Semmens B. X., Micheli F. (2012b). Assessing the effects of large mobile predators on ecosystem connectivity. Ecol. Appl. 22, 1711–1717. doi: 10.1890/11-1653.1
McClenachan L., Ferretti F., Baum J. K. (2012). From archives to conservation: Why historical data are needed to set baselines for marine animals and ecosystems. Conserv. Lett. 5, 349–359. doi: 10.1111/j.1755-263X.2012.00253.x
McConnell B. J., Chambers C., Nicholas K. S., Fedak M. A. (1992). Satellite tracking of grey seals (Halichoerus grypus). J. Zool. 226, 271–282. doi: 10.1111/j.1469-7998.1992.tb03839.x
McMahon C. R., Roquet F., Baudel S., Belbeoch M., Bestley S., Blight C., et al. (2021). Animal borne ocean sensors–AniBOS–An essential component of the global ocean observing system. Front. Mar. Sci. 8. doi: 10.3389/fmars.2021.751840
Micheli F., Halpern B. S., Walbridge S., Ciriaco S., Ferretti F., Fraschetti S., et al. (2013). Cumulative human impacts on Mediterranean and black sea marine ecosystems: assessing current pressures and opportunities. PloS One 8, e79889. doi: 10.1371/journal.pone.0079889
Miller V., Mirabal-Patterson A., García-Rodríguez E., Karr K., Whittle D. (2018). The SOS pesca project: A multinational and intersectoral collaboration for sustainable fisheries, Marine conservation and improved quality of life in coastal communities. Med. Rev. 20, 65–70. doi: 10.37757/MR2018.V20.N2.13
Moher D., Liberati A., Tetzlaff J., Altman D. G. (2009). Preferred reporting items for systematic reviews and meta-analyses: the PRISMA statement. Br. Med. J. 339, b2535–b2535. doi: 10.1136/bmj.b2535
Morais P., Afonso L., Dias E. (2021). Harnessing the power of social media to obtain biodiversity data about cetaceans in a poorly monitored area. Front. Mar. Sci. 8. doi: 10.3389/fmars.2021.765228
Moro S., Jona-Lasinio G., Block B., Micheli F., De Leo G., Serena F., et al. (2020). Abundance and distribution of the white shark in the Mediterranean Sea. Fish Fish. 21, 338–349. doi: 10.1111/faf.12432
Moullec F., Barrier N., Drira S., Guilhaumon F., Hattab T., Peck M. A., et al. (2022). Using species distribution models only may underestimate climate change impacts on future marine biodiversity. Ecol. Modell. 464, 109826. doi: 10.1016/j.ecolmodel.2021.109826
Myers R. A., Baum J. K., Shepherd T. D., Powers S. P., Peterson C. H. (2007). Cascading effects of the loss of apex predatory sharks from a coastal ocean. Science 315, 1846–1850. doi: 10.1126/science.1138657
Myers R. A., Worm B. (2003). Rapid worldwide depletion of predatory fish communities. Nature 423, 280–283. doi: 10.1038/nature01610
Navarro J., Louzao M., Igual J. M., Oro D., Delgado A., Arcos J. M., et al. (2009). Seasonal changes in the diet of a critically endangered seabird and the importance of trawling discards. Mar. Biol. 156, 2571–2578. doi: 10.1007/s00227-009-1281-3
Newsome S. D., Clementz M. T., Koch P. L. (2010). Using stable isotope biogeochemistry to study marine mammal ecology. Mar. Mammal Sci. 26, 509–572. doi: 10.1111/j.1748-7692.2009.00354.x
Newsome S. D., Martinez del Rio C., Bearhop S., Phillips D. L. (2007). A niche for isotopic ecology. Front. Ecol. Environ. 5, 429–436. doi: 10.1890/1540-9295(2007)5[429:ANFIE]2.0.CO;2
O’Dea R. E., Lagisz M., Jennions M. D., Koricheva J., Noble D. W. A., Parker T. H., et al. (2021). Preferred reporting items for systematic reviews and meta-analyses in ecology and evolutionary biology: a PRISMA extension. Biol. Rev. 96, 1695–1722. doi: 10.1111/brv.12721
O’Hagan A. M. (2020). “Ecosystem-based management (EBM) and ecosystem services in EU law, policy and governance,” in Ecosystem-based management, ecosystem services and aquatic biodiversity: Theory, tools and applications. Eds. O’Higgins T., Lago M., DeWitt T. H. (Springer, Amsterdam), 353–372.
Ollason J. C., Dunnet G. M. (1978). Age, experience and other factors affecting the breeding success of the fulmar, Fulmarus glacialis, in Orkney. J. Anim. Ecol. 47, 961–976. doi: 10.2307/3681
Oppel S., Meirinho A., Ramírez I., Gardner B., O’Connell A. F., Miller P. I., et al. (2012). Comparison of five modelling techniques to predict the spatial distribution and abundance of seabirds. Biol. Conserv. 156, 94–104. doi: 10.1016/j.biocon.2011.11.013
Oro D., Aguilar J. S., Igual J. M., Louzao M. (2004). Modelling demography and extinction risk in the endangered Balearic shearwater. Biol. Conserv. 116, 93–102. doi: 10.1016/S0006-3207(03)00180-0
Oro D., Genovart M., Tavecchia G., Fowler M. S., Martínez-Abraín A. (2013). Ecological and evolutionary implications of food subsidies from humans. Ecol. Lett. 16, 1501–1514. doi: 10.1111/ele.12187
Oro D., Martinez-Villalta A. (1992). The colony of the Audouin’s gull at the Ebro Delta. Avocetta 162, 98–101.
Ouled-Cheikh J., Ramírez F., Sánchez-Fortún M., Cortejana A., Sanpera C., Carrasco J. L. (2022). Fishing activities shape the flight behaviour of an opportunistic predator species. Estuarine Coast. Shelf Sci. 278, 108089. doi: 10.1016/j.ecss.2022.108089
Ouled-Cheikh J., Sanpera C., Becares J., Arcos J. M., Carrasco J. L., Ramírez F. (2020). Spatiotemporal analyses of tracking data reveal fine-scale, daily cycles in seabird–fisheries interactions. ICES J. Mar. Sci. 77, 2508–2517. doi: 10.1093/icesjms/fsaa098
Paleczny M., Hammill E., Karpouzi V., Pauly D. (2015). Population trend of the world’s monitored seabirds 1950-2010. PloS One 10, e0129342. doi: 10.1371/journal.pone.0129342
Papastamatiou Y. P., Mourier J., TinHan T., Luongo S., Hosoki S., Santana-Morales O., et al. (2022). Social dynamics and individual hunting tactics of white sharks revealed by biologging. Biol. Lett. 18, 20210599. doi: 10.1098/rsbl.2021.0599
Pauly D. (1995). Anecdotes and the shifting baseline syndrome of fisheries. Trends Ecol. Evol. 10 (10), 430. doi: 10.1016/S0169-5347(00)89171-5
Pauly D. (2000). “Global Change, Fisheries, and the Integrity of Marine Ecosystem: The Future Has Already Begun. Chapter 13,” in Ecological Integrity: Integrating Environment, Conservation and Health, vol. 2000 . Eds. Pimentel D., Westra L., Ross R. F. (Island Press, Washington, DC), 227–239.
Pauly D., Christensen V., Dalsgaard J., Froese R., Torres F. Jr., Torres F. (1998). Fishing down marine food webs. Science 279, 860–863. doi: 10.1126/science.279.5352.860
Payo-Payo A., Sanz-Aguilar A., Genovart M., Bertolero A., Piccardo J., Camps D., et al. (2018). Predator arrival elicits differential dispersal, change in age structure and reproductive performance in a prey population. Sci. Rep. 8, 1971. doi: 10.1038/s41598-018-20333-0
Peck M. A., Arvanitidis C., Butenschön M., Canu M. D., Chatzinikolaou E., Cucco A., et al. (2018). Projecting changes in the distribution and productivity of living marine resources: A critical review of the suite of modeling approaches used in the large European project VECTORS. Estuar. Coast. Shelf Sci. 201, 40–55. doi: 10.1016/j.ecss.2016.05.019
Pennino M. G., Conesa D., Lopez-Quilez A., Munoz F., Fernández A., Bellido J. M. (2016). Fishery-dependent and -independent data lead to consistent estimations of essential habitats. ICES J. Mar. Sci. 73, 2302–2310. doi: 10.1093/icesjms/fsw062
Pennino M. G., Muñoz F., Conesa D., López-Quίlez A., Bellido J. M. (2013). Modeling sensitive elasmobranch habitats. J. Sea Res. 83, 209–218. doi: 10.1016/j.seares.2013.03.005
Pérez-Jorge S., Gomes I., Hayes K., Corti G., Louzao M., Genovart M., et al. (2016). Effects of nature-based tourism and environmental drivers on the demography of a small dolphin population. Biol. Conserv. 197, 200–208. doi: 10.1016/j.biocon.2016.03.006
Pérez-Roda A., Delord K., Boué A., Arcos J. M., García D., Micol T., et al. (2017). Identifying Important Atlantic Areas for the conservation of Balearic shearwaters: Spatial overlap with conservation areas. Deep Sea Res. Part II: Topical Stud. Oceanogr. 141, 285–293. doi: 10.1016/j.dsr2.2016.11.011
Phillips R. A., Gales R., Baker G. B., Double M. C., Favero M., Quintana F., et al. (2016). The conservation status and priorities for albatrosses and large petrels. Biol. Conserv. 201, 169–183. doi: doi: 10.1016/j.biocon.2016.06.017
Pikitch E. K., Santora C., Babcock E. A., Bakun A., Bonfil R., Conover D. O., et al. (2004). Ecosystem-based fishery management. Science 305, 346–347. doi: 10.1126/science.1098222
Pires R., Neves H. C. (2001). Mediterranean monk seal Monachus monachus conservation: A case study in the Desertas Islands. Mammalia 65, 301–308. doi: 10.1515/mamm.2001.65.3.301
Plagányi É. (2007). Models for an Ecosystem Approach to Fisheries (Rome, Italy: Food and Agriculture Organization).
Plagányi É.E., Punt A. E., Hillary R., Morello E. B., Thébaud O., Hutton T., et al. (2012). Multispecies fisheries management and conservation: tactical applications using models of intermediate complexity. Fish Fish. 15, 1–22. doi: 10.1111/j.1467-2979.2012.00488.x
Postaire B. D., Bakker J., Gardiner J., Wiley T. R., Chapman D. D. (2020). Environmental DNA detection tracks established seasonal occurrence of blacktip sharks (Carcharhinus limbatus) in a semi-enclosed subtropical bay. Sci. Rep. 10, 11847. doi: 10.1038/s41598-020-68843-0
Pressey R. L., Bottrill M. C. (2009). Approaches to landscape-and seascape-scale conservation planning: convergence, contrasts and challenges. Oryx 43, 464–475. doi: 10.1017/S0030605309990500
Punt A. E., Donovan G. P. (2007). Developing management procedures that are robust to uncertainty: lessons from the International Whaling Commission. ICES J. Mar. Sci. 64, 603–612. doi: 10.1093/icesjms/fsm035
Queirós A., Huebert K., Keyl F., Fernandes J., Stolte W., Maar M., et al. (2016). Solutions for ecosystem-level protection of ocean systems against climate change. Glob. Change Biol. 22, 3927–3936. doi: 10.1111/gcb.13423
Ramírez F., Afán I., Bouten W., Carrasco J. L., Forero M. G., Navarro J. (2020). Humans shape the year-round distribution and habitat use of an opportunistic scavenger. Ecol. Evol. 10, 4716–4725. doi: 10.1002/ece3.6226
Ramírez-León M. R., García-Aguilar M. C., Romo-Curiel A. E., Ramírez-Mendoza Z., Fajardo-Yamamoto A., Sosa-Nishizaki O. (2021). Habitat suitability of cetaceans in the Gulf of Mexico using an ecological niche modelling approach. PeerJ. Mar. 17, e10834. doi: 10.7717/peerj.10834
Ramos R., González-Solís J. (2012). Trace me if you can: the use of intrinsic biogeochemical markers in marine top predators. Front. Ecol. Envir. 10, 258–266. doi: 10.1890/110140
Ramos R., Ramírez F., Sanpera C., Jover L., Ruiz X. (2009). Feeding ecology of yellow-legged gulls Larus michahellis in the western Mediterranean: a comparative assessment using conventional and isotopic methods. Mar. Ecol.Prog. Ser. 377, 289–297. doi: 10.3354/meps07792
Rechsteiner E. U., Rosen D. A., Trites A. W. (2013). Energy requirements of Pacific white-sided dolphins (Lagenorhynchus obliquidens) as predicted by a bioenergetic model. J. Mammal. 94, 820–832. doi: 10.1644/12-MAMM-A-206.1
Reijnders P. J. H. (1992). Retrospective population analysis and related future management perspectives for the harbour seal Phoca vitulina in the Wadden Sea Vol. 20 (Netherlands Institute for Sea Research, Publication Series), 193–197.
Reijnders P. J. H., van Dijk J., Kuiper D. (1995). Recolonization of the Dutch Wadden Sea by the grey seal Halichoerus grypus. Biol. Conserv. 71, 231–235. doi: 10.1016/0006-3207(94)00032-L
Ripple W. J., Wolf C., Newsome T. M., Betts M. G., Ceballos G., Courchamp F., et al. (2019). Are we eating the world’s megafauna to extinction? Conserv. Lett. 12, e12627. doi: 10.1111/conl.12627
Robbins J. R., Babey L., Embling C. B. (2020). Citizen science in the marine environment: Estimating common dolphin densities in the northeast Atlantic. PeerJ 8, e8335. doi: 10.7717/peerj.8335
Rodríguez A., Arcos J. M., Bretagnolle V., Dias M. P., Holmes N. D., Louzao M., et al. (2019). Future directions in conservation research on petrels and shearwaters. Front. Mar. Sci. 6. doi: 10.3389/fmars.2019.00094
Roman J., McCarthy J. J. (2010). The whale pump: marine mammals enhance primary productivity in a coastal basin. PloS One 5, e13255. doi: 10.1371/journal.pone.0013255
Romero M. A., Coscarella M. A., Adams G. D., Pedraza J. C., González R. A., Crespo E. A. (2022). Historical reconstruction of the population dynamics of southern right whales in the southwestern Atlantic Ocean. Sci.Rep. 12, 1–17. doi: 10.1038/s41598-022-07370-6
Ropert-Coudert Y., Wilson R. P. (2005). Trends and perspectives in animal-attached remote sensing. Front. Ecol. Environ. 3, 437–444. doi: 10.1890/1540-9295(2005)003[0437:TAPIAR]2.0.CO;2
Rosen D. A., Trites A. W. (2000). Pollock and the decline of Steller sea lions: testing the junk-food hypothesis. Can. J. Zoo. 78, 1243–1250. doi: 10.1139/z00-060
Russell D. J., Morris C. D., Duck C. D., Thompson D., Hiby L. (2019). Monitoring long-term changes in UK grey seal pup production. Aquat. Conserv.: Mar. Freshw. Ecosyst. 29, 24–39. doi: 10.1002/aqc.3100
Russell D. J. F., Wanless S., Collingham Y. C., Huntley B., Hamer C. K. (2015). Predicting future European breeding distributions of British seabird species under climate change and unlimited/no dispersal scenarios. Diversity 7, 342–359. doi: 10.3390/d7040342
Ryan P. G., Moore C. J., van Franeker J. A., Moloney C. L. (2009). Monitoring the abundance of plastic debris in the marine environment. Philos. Trans. R. Soc B: Biol. Sci. 364, 1999–2012. doi: 10.1098/rstb.2008.0207
Saavedra C., Gerrodette T., Louzao M., Valeiras J., Garcia S., Cervino S., et al. (2018). Assessing the environmental status of the short-beaked common dolphin (Delphinus delphis) in North-western Spanish waters using abundance trends and safe removal limits. Prog. Oceanogr. 166, 66–75. doi: 10.1016/j.pocean.2017.08.006
Sáenz-Arroyo A., Roberts C. M., Torre J., Cariño-Olvera M. (2005). Using fishers’ anecdotes, naturalists’ observations and grey literature to reassess marine species at risk: The case of the Gulf grouper in the Gulf of California, Mexico. Fish Fish. 6, 121–133. doi: 10.1111/j.1467-2979.2005.00185.x
Sbragaglia V., Espasandín L., Jarić I., Vardi R., Ramírez F., Coll M. (2024). Tracking ongoing transboundary marine distributional range shifts in the digital era. Mar. Ecol. Prog. Ser 728, 103-114. doi: 10.3354/meps14309
Sbragaglia V., Jolles J. W., Coll M., Arlinghaus. R. (2021). Fisheries-induced changes of shoaling behaviour: mechanisms and consequences. Trends Ecol. Evol. 36, 885–888. doi: 10.1016/j.tree.2021.06.015
Şekercioğlu C. H., Daily G. C., Ehrlich P. R. (2004). Ecosystem consequences of bird declines. PNAS 101 (52), 18042–18047. doi: 10.1073/pnas.0408049101
Sergio F., Schmitz O. J., Krebs C. J., Holt R. D., Heithaus M. R., Wirsing A. J., et al. (2014). Towards a cohesive, holistic view of top predation: a definition, synthesis and perspective. Oikos 123, 1234–1243. doi: 10.1111/oik.01468
Serpetti N., Benjamins S., Brain S., Collu M., Harvey B. J., Heymans J. J., et al. (2021). Modeling small scale impacts of multi-purpose platforms: an ecosystem approach. Front. Mar. Sci. 8. doi: 10.3389/fmars.2021.694013
Shabtay A., Lagabrielle E., Plot V., Potin G., Guyomard D. (2020). Marine spatial planning provides a comprehensive framework for building evidence-based shark risk management policies with sea-users. Environ. Sci. Policy 111, 18–26. doi: 10.1016/j.envsci.2020.05.014
Sherman C. S., Heupel M. R., Moore S. K., Chin A., Simpfendorfer C. A. (2020). When sharks are away, rays will play: effects of top predator removal in coral reef ecosystems. Mar. Ecol. Prog. Ser. 641, 145–157. doi: 10.3354/meps13307
Spalding M. D., Fox H. E., Allen G. R., Davidson N., Ferdaña Z. A., Finlayson M. A. X., et al. (2007). Marine ecoregions of the world: a bioregionalization of coastal and shelf areas. BioScience 57, 573–583. doi: 10.1641/B570707
Spedicato M. T., Massutí E., Mérigot B., Tserpes G., Jadaud A., Relini G. (2019). The MEDITS trawl survey specifications in an ecosystem approach to fishery management. Sci. Mar. 83S1, 9–20. doi: 10.3989/scimar.05015.06A
Speed C. W., Rees M. J., Cure K., Vaughan B., Meekan M. G. (2019). Protection from illegal fishing and shark recovery restructures mesopredatory fish communities on a coral reef. Ecol. Evol. 9, 10553–10566. doi: 10.1002/ece3.5575
Steenbeek J., Coll M., Gurney L., Melin F., Hoepffner N., Buszowski J., et al. (2013). Bridging the gap between ecosystem modeling tools and geographic information systems: Driving a food web model with external spatial-temporal data. Ecol. Modell. 263, 139–151. doi: 10.1016/j.ecolmodel.2013.04.027
Stelzenmüller V., Coll M., Cormier R., Mazaris A. D., Pascual M., Loiseau C., et al. (2020). Operationalizing risk-based cumulative effect assessments in the marine environment. Sci. Total Environ. 724, 138118. doi: 10.1016/j.scitotenv.2020.138118
Stelzenmüller V., Coll M., Mazaris A. D., Giakoumi S., Katsanevakis S., Portman M. E., et al. (2018). A risk-based approach to cumulative effect assessments for marine management. Sci. Total Environ. 612, 1132–1140. doi: 10.1016/j.scitotenv.2017.08.289
Stevens J. D., Bonfil R., Dulvy N. K., Walker P. A. (2000). The effects of fishing on sharks, rays, and chimaeras (Chondrichthyans), and the implications for marine ecosystems. ICES J. Mar. Sci. 57, 476–494. doi: 10.1006/jmsc.2000.0724
Stock B. C., Jackson A. L., Ward E. J., Parnell A. C., Phillips D. L., Semmens B. X. (2018). Analysing mixing systems using a new generation of Bayesian tracer mixing models. PeerJ 6, e5096. doi: 10.7717/peerj.5096
Suarez-Bregua P., Álvarez-González M., Parsons K. M., Rotllant J., Pierce G. J., Saavedra C. (2022). Environmental DNA (eDNA) for monitoring marine mammals: Challenges and opportunities. Front. Mar. Sci. 9. doi: 10.3389/fmars.2022.987774
Sulikowski J. A., Hammerschlag N. (2023). A novel intrauterine satellite transmitter to identify parturition in large sharks. Sci. Adv. 9, eadd6340. doi: 10.1126/sciadv.add6340
Tavecchia G., Minguez E., De León A., Louzao M., Oro D. (2008). Living close, doing differently: small-scale asynchrony in demography of two species of seabirds. Ecology 89, 77–85. doi: 10.1890/06-0326.1
Terborgh J., Estes J. A. (2013). Trophic Cascades: Predators, Prey, and the Changing Dynamics of Nature (Washington, DC: Island Press).
Tikhonov G., Opedal Ø.H., Abrego N., Lehikoinen A., de Jonge M. M., Oksanen J., et al. (2020). Joint species distribution modelling with the r-package Hmsc. Methods Ecol. Evol. 11, 442–447. doi: 10.1111/2041-210X.13345
Tittensor D. P., Eddy T. D., Lotze H. K., Galbraith E. D., Cheung W., Barange M., et al. (2018). A protocol for the intercomparison of marine fishery and ecosystem models: Fish-MIP v1.0. Geosci. Model. Dev. 11, 1421–1442. doi: 10.5194/gmd-11-1421-2018
Tittensor D. P., Novaglio C., Harrison C. S., Heneghan R. F., Barrier N., Bianchi D., et al. (2021). Next-generation Earth System Models reveal higher climate risks for marine ecosystems. Nat. Clim. Change 11, 973–981. doi: 10.1038/s41558-021-01173-9
Tsikliras A. C., Dinouli A., Tsiros V.-Z., Tsalkou E. (2015). The Mediterranean and black sea fisheries at risk from overexploitation. PloS One 10, e0121188. doi: 10.1371/journal.pone.0121188
Tsirintanis K., Azzurro E., Crocetta F., Dimiza M., Froglia C., Gerovasileiou V., et al. (2022). Bioinvasion impacts on biodiversity, ecosystem services, and human health in the Mediterranean Sea. Aquat. Invasions 17 (3), 308–352. doi: 10.3391/ai.2022.17.3.01
UNGA (United Nations General Assembly) (1990a). Resolution on large-scale pelagic driftnet fishing and its impact on the living marine resources of the world’s oceans and seas. G.A. Res. 44/225, 29 I.L.M. 1555 (1990). United Nations, NY, USA. doi: 10.1017/S0020782900016879
UNGA (United Nations General Assembly) (1990b). Resolution on large-scale pelagic driftnet fishing and its impact on the living marine resources of the world’s oceans and seas G.A. Res. 45/197, 29 I.L.M. 1449 (1990).United Nations, NY, USA.
UNGA (United Nations General Assembly) (1992). Resolution on Large-Scale Pelagic Drift-net Fishing and its Impact on the Living Marine Resources of the World’s Oceans and Seas, G.A. Res. 46/215, 31 I.L.M. 241 (1992).United Nations, NY, USA.
Valsecchi E., Tavecchia G., Boldrocchi G., Boldrocci G., Coppola E., Ramella D., et al. (2023). Playing “hide and seek” with the Mediterranean monk seal: a citizen science dataset reveals its distribution from molecular traces (eDNA). Sci. Rep. 13, 2610. doi: 10.1038/s41598-023-27835-6
Verborgh P., Gauffier P., Brévart C., Giménez J., Esteban R., Carbou M., et al. (2019). Epizootic effect and aftermath in a pilot whale population. Aquat. Conserv.: Mar. Freshw. Ecosyst. 29, 820–828. doi: 10.1002/aqc.3082
Waggitt J. J., Evans P. G., Andrade J., Banks A. N., Boisseau O., Bolton M., et al. (2020). Distribution maps of cetacean and seabird populations in the North-East Atlantic. J. Appl. Ecol. 57, 253–269. doi: 10.1111/1365-2664.13525
Walker K. A., Trites A. W., Haulena M., Weary D. M. (2012). A review of the effects of different marking and tagging techniques on marine mammals. Wildlife Res. 39, 15–30. doi: 10.1071/WR10177
Walsh W. A., Bigelow K. A., Sender K. L. (2009). Decreases in shark catches and mortality in the Hawaii-based longline fishery as documented by fishery observers. Mar. Coast. fish. 1, 270–282. doi: 10.1577/C09-003.1
Watanabe Y. Y., Papastamatiou Y. P. (2023). Biologging and biotelemetry: tools for understanding the lives and environments of marine animals. Annu. Rev. Anim. Biosci. 11, 247–267. doi: 10.1146/annurev-animal-050322-073657
Watanabe Y. Y., Takahashi A. (2013). Linking animal-borne video to accelerometers reveals prey capture variability. Proc. Natl. Acad. Sci. U.S.A. 110, 2199–2204. doi: 10.1073/pnas.1216244110
Weimerskirch H., Louzao M., de Grissac S., Delord K. (2012). Changes in wind pattern alter albatross distribution and life-history traits. Science 335, 211–214. doi: 10.1126/science.1210270
Westell A., Sakai T., Valtierra R., Van Parijs S. M., Cholewiak D., DeAngelis A. (2022). Sperm whale acoustic abundance and dive behaviour in the western North Atlantic. Sci. Rep. 12, 16821. doi: 10.1038/s41598-022-20868-3
Whiteman J. P., Elliott Smith E. A., Besser A. C., Newsome S. D. (2019). A guide to using compound-specific stable isotope analysis to study the fates of molecules in organisms and ecosystems. Diversity 11, 8. doi: 10.3390/d11010008
Wiig Ø., Aars J., Belikov S. E., Boltunov A. (2007) Ursus maritimus (Europe assessment). The IUCN Red List of Threatened Species 2007 (Accessed 03 July 2023).
Wiig Ø., Amstrup S., Atwood T., Laidre K., Lunn N., Obbard M., et al. (2015) Ursus maritimus. The IUCN Red List of Threatened Species 2015 (Accessed 03 July 2023).
Winship A. J., Trites A. W., Rosen D. A. (2002). A bioenergetic model for estimating the food requirements of Steller sea lions Eumetopias jubatus in Alaska, USA. Mar. Ecol. Prog. Ser. 229, 291–312. doi: 10.3354/meps229291
Keywords: top predators, monitoring, ecosystem-based management, ecosystem modelling approach, population trend estimation
Citation: Fortuna CM, Fortibuoni T, Bueno-Pardo J, Coll M, Franco A, Giménez J, Stranga Y, Peck MA, Claver C, Brasseur S, Fernández-Corredor E, Fraschetti S, Garcia-Garin O, van Leeuwen A, Louzao M, Pedrajas A, Raicevich S, Ramírez F, Ransijn J, Russell D, Serena F, Sbragaglia V and Katsanevakis S (2024) Top predator status and trends: ecological implications, monitoring and mitigation strategies to promote ecosystem-based management. Front. Mar. Sci. 11:1282091. doi: 10.3389/fmars.2024.1282091
Received: 23 August 2023; Accepted: 26 February 2024;
Published: 13 May 2024.
Edited by:
Alberto Basset, University of Salento, ItalyReviewed by:
Catarina Eira, University of Aveiro, PortugalFelipe Galván-Magaña, Centro Interdisciplinario de Ciencias Marinas (IPN), Mexico
Copyright © 2024 Fortuna, Fortibuoni, Bueno-Pardo, Coll, Franco, Giménez, Stranga, Peck, Claver, Brasseur, Fernández-Corredor, Fraschetti, Garcia-Garin, van Leeuwen, Louzao, Pedrajas, Raicevich, Ramírez, Ransijn, Russell, Serena, Sbragaglia and Katsanevakis. This is an open-access article distributed under the terms of the Creative Commons Attribution License (CC BY). The use, distribution or reproduction in other forums is permitted, provided the original author(s) and the copyright owner(s) are credited and that the original publication in this journal is cited, in accordance with accepted academic practice. No use, distribution or reproduction is permitted which does not comply with these terms.
*Correspondence: Caterina Maria Fortuna, caterina.fortuna@isprambiente.it